Introduction
Sport-specific performance is a complex, multi-dimensional concept crucial for success in competitive sports (Ford et al., 2011; Johnston et al., 2018). It is characterized by a unique blend of physical, technical, tactical, and psychological elements, each carefully tailored to the specific demands of a particular sport. This performance extends beyond basic physical attributes like strength, power, endurance, speed, agility, and flexibility (Tucker and Collins, 2012). It also includes mastering specialized movement patterns that are essential for effectively executing tasks unique to a sport (Elferink-Gemser et al., 2007). For example, the performance of a kick in soccer (Katis et al., 2013) or a serve in tennis (Etnyre, 1998) requires not just force but also accuracy, control, and decision-making. It is important to note that researching sport-specific performance entails significant challenges, primarily due to the intricacies involved in identifying key aspects or predictors of success within competitive sports. Direct evidence establishing predictors of competition success is scarce. The methodological constraints are particularly pronounced in dynamic and multifaceted sports disciplines, where capturing the essence of sport-specific performance is both critical and challenging (Chaabene et al., 2018). These challenges can be magnified in team sports where, outside of the attributes that a given athlete may possess or even technical and tactical components, dynamics of communication and interpersonal relationships can influence competitive outcomes. Despite these difficulties, in some systematic reviews, researchers have attempted to identify and evaluate sport-specific outcomes (Chaabene et al., 2018; Saeterbakken et al., 2022; Thiele et al., 2020).
Elite athletes compete at the highest levels of national and international sports. Their development in sports performance goes beyond innate talent to include unique training methods designed specifically for their advanced needs, which often differ from those of non-elite athletes (Lorenz et al., 2013). Defining the criteria to classify 'elite' athletes has been a challenging task for researchers, which has resulted in confusion and inconsistency in the literature. Although some attempts have been made to clarify the issue, as seen in Swann et al. (2015), there remains a considerable lack of consensus among researchers regarding this matter. Because of this, the term 'elite' in sports research spans a wide range, from athletes with only few years of training experience, to academy or university competitors, top-ranked participants, skilled national or international competitors, and extends to professionals, semi-professionals, world-class athletes, medalists, Olympians, and even Olympic or world champions (Williams et al., 2017). This absence of a universally accepted standard for what makes an athlete ‘elite’ presents a challenge to the validity of research focusing on high-level sports performance and training methods tailored to these athletes. It complicates the process of drawing reliable conclusions about the unique training and performance characteristics that distinguish elite athletes from their non-elite counterparts. In this work, we address this challenge by categorizing elite athletes as those competing at the highest international or national levels in their respective sports.
Resistance training (RT) is widely acknowledged as a vital component in enhancing athletic performance, significantly influencing an athlete's sport-specific abilities and skills (Abdi et al., 2019; Loturco et al., 2024; Spieszny et al., 2022; Thiele et al., 2020b). Its diverse nature allows for a broad spectrum of benefits, adaptable to various athletic needs and goals (Harries et al., 2012; Loturco et al., 2023a; Saeterbakken et al., 2022). This training method encompasses a wide range of tools, including free weights, machines, resistance bands, plyometrics, resisted sprint training, core stability exercises, and bodyweight exercises (Loturco et al., 2023b; Morris et al., 2022; Stone et al., 2000). The selection of tools and techniques can be specifically tailored to enhance particular muscle groups (Schoenfeld et al., 2019), improve overall strength, increase power or build endurance, depending on the athlete’s sport and individual requirements. However, this diversity also poses a challenge in identifying the most effective training regimen for each athlete, requiring a deep understanding of the sport's specific physical demands and the athlete’s personal performance goals (Harries et al., 2012; Thiele et al., 2020). For example, a basketball player may focus on plyometric exercises to improve explosive power and agility, vital for jumping and quick movements on the court. In contrast, a swimmer may utilize resistance bands to strengthen upper body muscles, crucial for effective stroke techniques in the water, and can deliberately avoid repetition ranges thought to be more effective at inducing muscle hypertrophy in an effort, to prevent an increase in their cross-sectional area.
In this systematic review, we address a critical gap in the current understanding of how RT specifically benefits elite athletes. Existing research predominantly focuses on the general physical advantages of RT, such as enhanced muscle mass and overall strength (Naclerio et al., 2013; Ratel, 2011; Zouita et al., 2023). However, when sport-specific performance is examined, participants are often not elite athletes (Harries et al., 2012), or studies combine data from elite athletes with those of sub-elite and recreational athletes, leading to less distinct findings (Thiele et al., 2020). This situation results in a lack of clarity on how adaptations derived from RT translate into enhanced sport-specific performance, particularly at the elite level. Our review aimed to synthesize available evidence to discern the specific impact of various RT methods on sport-specific skills and performance of elite athletes. The objective was twofold: firstly, to systematically analyze and integrate findings from existing studies, thereby establishing a clear understanding of the role of RT in elite sports performance. Secondly, the review sought to provide evidence-based recommendations for optimizing RT protocols for elite athletes, identifying strategies that would effectively enhance athletic performance. Such a focused approach is crucial for advancing the field of sports science and guiding coaches toward evidence-based practices in the development of elite-level athletes.
Methods
Search Strategy
This systematic review was conducted following the PRISMA guidelines (Moher et al., 2009). Given that the study did not entail direct involvement of human subjects, the institutional review board approval was not required. An extensive search was conducted across four electronic databases (PubMed, Scopus, SPORTDiscus, and Web of Science) using specific search terms combined with Boolean operators, as detailed in Table 1. This search included all publications available up to November 19, 2023, and was not limited by language.
Table 1
Searched terms used to identify potential studies.
Study Selection and Data Extraction
During the study selection and data extraction phase, titles, abstracts, and full texts were systematically evaluated by two independent reviewers (H.M., M.S.) according to the PICO criteria outlined in Table 2. The EndNote X9.3.3 software (Clarivate Analytics) was used to facilitate the removal of duplicate articles, which was complemented by a manual check to ensure thorough de-duplication. Any discrepancies encountered in the selection process were resolved through discussion until a consensus was reached.
Table 2
Selection criteria.
Each study was coded for the following data: authors, sport, a competitive level (divided into categories: international level, national top-level), an intervention or a control group (resistance methods, e.g., strength training, power training, plyometrics, sprint resistance, trunk muscle training), the number of participants, sex, age, duration and frequency of intervention, the type of the performance test, outcomes (divided into categories: global, e.g., throwing velocity and local, e.g., running time). According to Thiele et al. (2020), multiple outcomes were ranked based on their significance for sport-specific performance, and the variable with the highest ranking was included in the subsequent analysis. Additionally, the types of resistance interventions were divided into two distinct categories: heavy and light (or power) load RT. Heavy RT typically involved lifting heavier weights (> 65% of one repetition maximum (1RM)) at a lower volume to increase muscle strength and hypertrophy, while light RT emphasized lifting lighter weights (< 60% of 1 RM) at a higher speed to enhance muscle power and speed of movement (Fisher et al., 2017). When study estimates were reported only graphically, data were extracted using ImageJ software version 1.53 (National Institutes of Health, USA, http://imagej.nih.gov).
Study Quality
Two independent reviewers (M.S. and P.T.) assessed the risk of bias and methodological quality of eligible articles using the PEDro scale (Maher et al., 2003). Discrepancies in PEDro scores were resolved through consultation with a third, independent, PEDro-certified assessor (H.M.). Compliance with the PEDro scale's criteria was indicated by "yes" for criteria met and "no" for the unmet ones, following the guidelines provided by PEDro (see https://pedro.org.au/english/resources/pedro-scale/). The PEDro scale evaluates internal validity and the presence of statistically replicable information, using a scale from 0 (high risk of bias) to 10 (low risk of bias). A score of 6 or more represents the threshold for studies with a low risk of bias. Item 1 only pertains to external validity and is not included in the calculation of the overall PEDro score. In training intervention studies, blinding participants to the exercise program is impossible, and often, it is also not feasible to blind the investigators. Therefore, items 5 and 6 of the PEDro scale, which are related to blinding, were removed, reducing the maximum score to 8. Following the criteria of previous exercise intervention reviews (Kümmel et al., 2016; Saeterbakken et al., 2022; Starzak et al., 2024), studies were categorized as follows: 6–8 excellent quality, 5 good quality, 4 moderate quality, and 0–3 poor quality. Points were awarded only when a study explicitly met the criteria. Additionally, the PEDro scale was modified for this study to align more closely with the specific methodological aspects of the strength and conditioning field (Makaruk et al., 2022).
Statistical Analysis
Between-subject standardized mean differences (SMDs) were calculated to determine the effects of RT on sport-specific performance, utilizing the formula: SMD = (mean1 − mean2) / spooled, where 'mean1' was the mean pre/post-test value of the intervention group, 'mean2' was the mean pre/post-test value of the control group, and 'spooled' was the pooled standard deviation (Saeterbakken et al., 2022). The SMD was adjusted for sample size according to Hedges and Olkin (2014), using the factor (1 − (3 / (4N − 9))), with 'N' representing the total sample size. Adjusted SMD values, calculated as the difference between pre-test and post-test SMDs, were also determined (Durlak, 2009). A random effects model was applied to weigh each study according to its standard error and to aggregate the weighted mean adjusted SMDs. Positive SMD values were consistently reported when RT was found to have a favorable effect compared with controls. A p value of < 0.05 was considered indicative of statistical significance. SMD values were categorized as trivial (< 0.2), small (0.2 ≤ SMD < 0.5), medium (0.5 ≤ SMD < 0.8), or large (SMD ≥ 0.8) (Cohen, 2013).
The inclusion of studies in the meta-analysis required a minimum of two intervention groups. Subject-related moderator variables such as the performance level, the type of sport-specific outcomes, sex, and the type of the resistance method were examined through sub-group analyses. Meta-analyses were conducted using Review Manager (RevMan5.3, Copenhagen: The Nordic Cochrane Centre, The Cochrane Collaboration, 2014). The level of agreement between reviewers was assessed using Kappa correlation coefficients (Altman, 1990). These coefficients are generally interpreted as follows: 0.81–1.00 indicates very good agreement, 0.61–0.80 good, 0.41–0.60 moderate, 0.21–0.40 fair, and < 0.20 poor (Altman, 1990). The I2 statistic was utilized to assess the level of between-study heterogeneity (Higgins et al., 2003), with values of 25%, 50%, and 75% corresponding to low, moderate, and high heterogeneity, respectively. Values exceeding 75% were deemed highly heterogeneous. Additionally, the chi-square test was used to ascertain whether analysis results were due to chance, with significant results indicated by low p-values or high chi-square statistics relative to degrees of freedom (df).
Results
Study Selection
A primary search of the electronic databases yielded 3607 studies. Additional three records were identified from other sources. After removing duplicates using Endnote software, and screening the titles and abstracts, 140 studies were selected for full-text revision. Five additional studies were found through a reference list search of eligible literature. Following all screening processes, 35 studies with 777 participants were included for qualitative analysis, and 29 studies were used for the meta-analysis. The flow of studies through the review process is reported in Figure 1.
Methodological Quality
Table 3 provides a detailed analysis of the methodological quality of the studies using the PEDro scale. The median quality score was 4 points, with a range from 3 to 6 points, indicating moderate methodological quality. The agreement rate between the assessments performed by the two reviewers was classified as very good since the Kappa correlation coefficient was 0.94.
Table 3
PEDro methodological quality rating scores.
[i] A detailed explanation of each item on the PEDro scale is available at https://pedro.org.au/english/resources/pedro-scale/.
None of the studies met the criterion of allocation concealment, with only one study explicitly stating whether an 'intention-to-treat' analysis was performed for the relevant outcomes. Moreover, except for one study, none of the studies included provided information on the criteria used for blinding methods.
Study Characteristics
The pooled number of participants across the studies was 777, with a sample size ranging from 9 to 36 participants (Table 4). Twenty-five studies involved only male participants, two studies included only female athletes, and eight studies combined male and female participants. The participants' average age varied from 13.2 to 29.6 years. Out of the total, twenty-nine studies enrolled athletes who were adults (aged ≥ 18 years), whereas six studies involved youth athletes (aged ≤ 18 years). In twenty-six studies, athletes were categorized as national elites, while in nine studies, they were categorized as international elite athletes.
Table 4a
Study characteristics.
Study | Population | Intervention | Training period/ Frequency | Outcome | |
---|---|---|---|---|---|
Sport/ Competitive level | Sex, sample size (n), age (years ±SD) | ||||
Aagaard et al. (2011) | Cycling/ International Elite | M, 19.5 (±0.8), EG: 7 CON: 7 | EG: weighted strength training, CON: regular training | 16 weeks, 2–3 sessions/week | 45-min endurance test, capacity (W), EG1↑ > E2↑ |
Ben Brahim et al. (2021) | Soccer/ International Elite | M, 18.8 (±0.8), EG: 20 CON: 14 | EG: resisted sprint training, CON: regular training | 6 weeks, 2 sessions/week | Ball-shooting speed (km•h−1), EG↑ > CON↑ |
Blazevich and Jenkins (2002) | Sprinters/ National Elite | M, 19.0 (±1.4), EG1: 5 EG2: 4 | EG1: strength training with high movement speeds, EG2: strength training with low movement speeds | 7 weeks, 2 sessions/week | 20-m acceleration (s), EG1↑ = EG2 |
Born et al. (2020) | Swimming/ International Elite | M&F, EG1: 10, 17.1 (±2.6), EG2: 11, 17.1 (±2.7) | EG1: heavy strength training, EG2: plyometric training | 6 weeks, 2 sessions/week | 25-m Freestyle swimming sprint (s), EG1 = EG2 |
Büsch et al. (2015) | Handball/ National Elite | M, EG1: 10, 16.7 (±0.6), EG2: 9, 17.4 (±0.9) | EG1: resistance training on stable ground, EG2: resistance training on unstable ground | 10 weeks, 2 sessions/week | Figure eight run performance (s), EG1↑ = EG2↑ |
Carlsson et al. (2017) | Cross country skiing/ National Elite | M&F, EG1: 14, M: 18.4 (±1.0), F: 18.5 (±0.8), EG2: 19, M: 18.4 (±0.9), F: 17.7 (±0.9) | EG1: strength training, EG2: ski-ergometer training | 6 weeks, 2 sessions/week | Maximal-speed test with the double-poling technique, maximal speed (km•h−1), EG1↑ = EG2↑ |
Cherif et al. (2022) | Handball/ National Elite | M, 22.1 (±3.0), EG: 11 CON: 11 | EG: strength and power training, CON: regular training | 12 weeks, 2 sessions/week | Throwing velocity (m·s−1), EG↑ = CON |
Fernandez-Fernandez et al. (2013) | Tennis/ National Elite | M, EG: 15, 13.2 (±0.6) CON: 15, 13.2 (±0.5) | EG: resistance training, CON: regular training | 6 weeks, 3 sessions/week | Serve velocity (km•h−1), EG↑ = CON |
Hermassi et al. (2010) | Handball/ National Elite | M, EG1: 9, 20.0 (±0.5) EG2: 9, 20.1 (±0.6) CON: 8, 20.0 (±0.7) | EG1: heavy strength training, EG2: moderate strength training, CON: regular training | 10 weeks, 2 sessions/week | Throwing velocity with run-up (m·s−1), EG1↑, EG2 > CON |
Hermassi et al. (2015) | Handball/ National Elite | M, EG1: 10, 18.4 (±0.5) EG2: 12, 18.7 (±0.5) CON: 12, 18.5 (±0.5) | EG1: resistance training, EG2: throwing training, CON: regular training | 8 weeks, 3 sessions/week | Throwing velocity with run-up (m·s−1), EG1↑ > CON; EG2 = CON |
Table 4b
Study characteristics.
Study | Population | Intervention | Training period/ Frequency | Outcome | |
---|---|---|---|---|---|
Sport/ Competitive level | Sex, sample size (n), age (years ±SD) | ||||
Hermassi et al. (2019) | Handball/ National Elite | M EG: 11, 20.3 (±0.5) CON: 11, 20.1 (±0.5) | EG: weighted strength training, CON: regular training | 12 weeks, 2 sessions/week | Throwing velocity with run-up (m·s−1), EG↑ > CON |
Jones et al. (2018) | Swimming/ International Elite | M&F, EG1: 4M, 2F, 19.4 (±1.1) EG2: 6M, 18.9 (±0.9) | EG1: weighted strength training, EG2: ballistic training | 6 weeks, 3 sessions/week | Time to 5 m (s), EG1 = EG2 |
Karpiński et al. (2020) | Swimming/ National Elite | M, EG: 15, 20.2 (±1.2) CON: 15, 20.0 (±1.9) | EG: core muscle training, CON: regular training | 6 weeks, 3 sessions/week | 50-m swimming time (s), EG↑ = CON |
Kristiansen et al. (2023) | Sprint Kayaking/ National Elite | M, 18.6 (±4.1), F, 17.0 (±1.4), EG1: 14 EG2: 12 | EG1: strength training (improve), EG2: strength training (maintain) | 6 weeks, 3 sessions/week | 200-m kayak ergometer all-out test (s), EG1↑ = EG2 |
Kristoffersen et al. (2019) | Cycling/ National Elite | M&F, 29.6 (±0.6), EG1: 14 EG2: 6 | EG1: resistance sprint training, EG2: heavy strength training | 6 weeks, 2 sessions/week | 5-min all-out cycling, average power output (W·kg−1), EG1↑ = EG2↑ |
Losnegard et al. (2011) | Cross-country skiing/ National Elite | M&F, EG: 9, M, 21.2 (±2.5), F, 21.3 (±5.1), CON: 10, M, 20.8 (±2.5), F, 22.6 (±2.4) | EG: heavy strength training, CON: regular training | 12 weeks, 1–2 sessions/week | 1.3-km skate-rollerski performance (VO2 max), EG = CON |
McEvoy and Newton (1998) | Baseball/ International Elite | M, 24 (±4), EG: 9 CON: 9 | EG: ballistic weight training, CON: regular training | 10 weeks, 3 sessions/week/ every 2 weeks | Throwing speed (m·s−1), EG↑ > CON |
Millet et al. (2002) | Triathlon/ International Elite | M, EG: 7, 24.3 (±5.2) CON: 8, 21.4 (±2.1) | EG: concurrent heavy weight training, endurance-strength training, CON: regular training | 14 weeks, 2 sessions/week | 3000-m running at VΔ25%, running economy (mL•kg−1•km−1), EG > CON |
Newton and McEvoy (1994) | Baseball/ National Elite | M, 18.6 (±1.9), EG1: 8 EG2: 8 CON: 8 | EG1: ballistic training, EG2: weighted strength training, CON: regular training | 8 weeks, 2 sessions/week | Throwing speed (m·s−1), EG2↑ = EG1, CON |
Newton et al. (1999) | Volleyball/ National Elite | M, 19 (±2), EG1: 8 EG2: 8 | EG1: ballistic training, EG2: strength training | 8 weeks, 2 sessions/week | Three-step vertical jump (cm), EG1↑ > EG2 |
Paavolainen et al. (1999) | Cross-country running/ National Elite | M, EG1: 10, 23 (3), EG2: 8, 24 (±5), | EG1: sport-specific explosive strength training with high training volume, CON: sport-specific explosive strength training with low training volume | 9 weeks | 5-km time trial (min), EG1↑ > CON |
Table 4c
Study characteristics.
Study | Population | Intervention | Training period/ Frequency | Outcome | |
---|---|---|---|---|---|
Sport/ Competitive level | Sex, sample size (n), age (years ±SD) | ||||
Ramos Veliz et al. (2014) | Water polo/ National Elite | M, 20.4 (±5.1), EG: 16 CON: 11 | EG: strength training, CON: regular training | 18 weeks, 2 sessions/week | Throwing velocity (m·s−1), EG↑ = CON |
Ramos Veliz et al. (2015) | Water polo/ National Elite | F, 26.4 (±4.3), EG: 11 CON: 10 | EG: lower body strength and power training, CON: regular training | 16 weeks, 2 sessions/week | Throwing velocity (m·s−1), EG↑ = CON |
Rønnestad et al. (2012) | Nordic Combined/ International Elite | M, EG: 8, 19 (±2) CON: 9, 20 (±3) | EG: heavy strength training, CON: regular training without heavy strength training | 12 weeks, 2 sessions/week | 7.5-km rollerski time-trial performance (s), EG = CON |
Rønnestad et al. (2015) | Cycling/ International Elite | M, EG: 9, 19.1 (±1.7), CON: 7, 20.1 (±1.6) | EG: heavy strength training, CON: regular training | 10/15 weeks, 1–2 sessions/week | Wingate test (VO2max), EG = CON |
Rønnestad et al. (2017) | Cycling/ International Elite | M&F, EG: 10M, 2F, 19 (±2), CON: 6M, 2F, 20 (±2) | EG: heavy strength training, CON: regular training | 10 weeks, 2 sessions/week | 40-min all-out trial (VO2max) EG = CON |
Sabido et al. (2016) | Handball/ National Elite | M, EG1: 12, 17.1 (±0.6), EG2: 11, 17.4 (±0.5), CON: 5, 17.0 (±0.6) | EG1: bench press throw exercise - known loads, EG2: bench press throw exercise - unknown loads, CON: regular training | 4 weeks, 2 sessions/week | Jumping throw (km·h−1), EG2↑ = EG1, CON |
Saez de Villarreal et al. (2014) | Water polo/ National Elite | M, EG1: 10, 19.7 (±5.4), EG2: 9, 18.5 (±2.3) | EG1: dry land resistance training, EG2: in water resistance training | 6 weeks, 3 sessions/week | Throwing speed (km·h−1), EG1 = EG2 |
Saez de Villarreal et al. (2015a) | Water polo/ National Elite | M, 23.4 (±4.1), EG1: 10 EG2: 10 EG3: 10 | EG1: strength and plyometric training, EG2: in water strength training, EG3: plyometric training | 6 weeks, 3 sessions/week | Throwing speed (km·h−1), EG1↑ > EG3↑; EG1↑ = EG2↑ |
Saez de Villarreal et al. (2015b) | Soccer/ National Elite | M EG: 13, 15.3 (±0.3), CON: 13, 14.9 (±0.2) | EG: plyometric-sprint training, CON: regular training | 9 weeks, 2 sessions/week | Ball-shooting speed (km·h−1), EG↑ > CON |
Saunders et al. (2006) | Long and middle distance running/ National Elite | M, EG: 7, 23.4 (±3.2), CON: 8, 24.9 (±3.2) | EG: plyometric training, CON: regular training | 9 weeks, 2–3 sessions/week | Treadmill running test, running economy at 18 km•h−1, (VO2; L•min−1), EG↑ > CON |
Sedano et al. (2009) | Soccer/ National Elite | F, EG: 10, 22.8 (±2.1), CON: 10, 23.0 (±3.2) | EG: plyometric training, CON: regular training | 12 weeks, 3 sessions/week | Kicking speed (km·h−1), EG↑ > CON |
Table 4d
Study characteristics.
Study | Population | Intervention | Training period/ Frequency | Outcome | |
---|---|---|---|---|---|
Sport/ Competitive level | Sex, sample size (n), age (years ±SD) | ||||
Sedano et al. (2013) | Soccer/ National Elite | M, EG: 11, 18.4 (±1.1), CON: 11, 18.2 (±0.9) | EG: plyometric training, CON: regular training | 10 weeks, 3 sessions/week | Kicking speed (km·h−1), EG↑ > CON |
Therell et al. (2022) | Cross-country skiing/ National Elite | M&F, EG1: 12, 18.1 (±0.6), EG2: 12, 18.0 (±1.5) | EG1: static core muscle training, EG2: dynamic core muscle training | 9 weeks, 3 sessions/week | Roller ski treadmill test, energetic cost (J·kg−1·m−1), EG1 = EG2 |
Wang et al. (2022) | Volleyball/ National Elite | M, EG1: 6, 20.8 (±1.5), EG2: 6, 20.5 (±1.4), EG3: 6, 20.2 (±0.8) | EG1: high-load resistance training, EG2: low-load resistance training with blood flow restriction, EG3: high-load resistance training with blood flow restriction | 8 weeks, 3 sessions/week | Three footed takeoff vertical jump (cm), EG3↑ > EG2; EG↑ = EG1 |
The duration of RT interventions varied from four to eight weeks, with training frequencies ranging from one to three sessions per week. The studies included in the analysis assessed sport-specific performance using various RT methods such as heavy strength training, ballistic training, plyometrics, concurrent strength and endurance training, core muscle training or different modalities of RT. These modalities included strength training on a stable and an unstable surface, dry-land and in-water strength training, as well as strength training with blood flow restriction, or combined methods of RT, including strength and plyometric training.
Twenty-three studies conducted comparisons between an intervention group that followed RT and an active control group that mostly followed a regular training routine in a specific sport, which was similar to the intervention group. Four of the twenty-three studies included two experimental groups compared to control groups. In addition, ten studies compared two different RT interventions without a control group, while two others compared three RT interventions exclusively.
Main Analyses
The primary meta-analysis, encompassing 23 studies with 460 elite athletes, demonstrated that RT significantly enhanced sport-specific performance, evidenced by a SMD of 1.16 (95% CI: 0.65, 1.66), indicative of a large effect size (Figure 2). Despite the noted heterogeneity (I2 = 84%), the overall positive effect was statistically significant (Z = 4.48, p < 0.00001). Subsequent analyses distinguished between international and national elite athletes (Figure 3). International elite athletes did not exhibit a statistically significant (p > 0.05) improvement in performance (SMD = 0.29; 95% CI: −0.07, 0.64; I2 = 0%), in contrast to national elite athletes who saw a larger, significant (p < 0.00001) benefit from RT (SMD = 1.57; 95% CI: 0.90, 2.24; I2 = 87%). A further breakdown revealed differential responses based on the type of the sport-specific outcome (Figure 4). Global outcomes yielded a medium but non-significant SMD of 0.49 (95% CI: −0.11, 1.09; p > 0.05; I2 = 70%), whereas local outcomes showed a more pronounced, significant effect (SMD = 1.59; 95% CI: 0.88, 2.29; p < 0.00001) with high heterogeneity (I2 = 87%). The sex-based analysis included 18 studies on male athletes and 2 on female athletes (Figure 5). The SMD for males was significant at 1.02 (95% CI: 0.55, 1.49; p < 0.00001; I2 = 77%), while females, despite a larger SMD of 3.40 (95% CI: −0.47, 7.26), did not reach statistical significance (p = 0.08), with very high heterogeneity (I2 = 95%). Lastly, the meta-analysis (Figure 6) comparing heavy versus light RT across eight studies found no significant (p > 0.05) differences in athletic performance outcomes (SMD = −0.03; 95% CI: −0.38, 0.31), and homogeneity in effects was observed (I2 = 0%).
Figure 2
The effects of resistance training (RT) compared with a control group (CON) on sport-specific performance in elite athletes. Experimental = experimental group, Control = control group, SMD = standardized mean difference, SE = standard error, Total = number of participants, CI = confidence interval, IV = inverse variance, Random = random effect model, df = degrees of freedom.
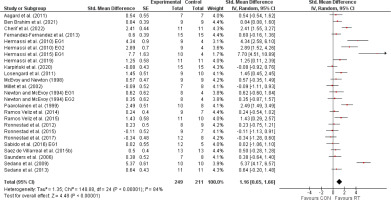
Figure 3
The effects of resistance training (RT) compared with a control group (CON) on sport-specific performance in elite athletes, delineated by the competitive level (international elite athletes, national elite athletes). Experimental = experimental group, Control = control group, SMD = standardized mean difference, SE = standard error, Total = number of participants, CI = confidence interval, IV = inverse variance, Random = random effect model, df = degrees of freedom.
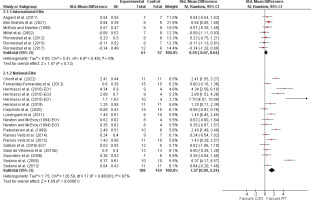
Figure 4
The effects of resistance training (RT) compared with a control group (CON) on sport-specific performance, categorized into global and local outcomes, in elite athletes.
Experimental = experimental group, Control = control group, SMD = standardized mean difference, SE = standard error, Total = number of participants, CI = confidence interval, IV = inverse variance, Random = random effect model, df = degrees of freedom.
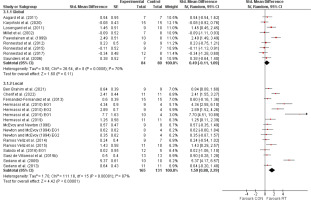
Figure 5
The effects of resistance training (RT) compared with a control group (CON) on sport-specific performance in elite athletes with a focus on sex-based differences.
Experimental = experimental group, Control = control group, SMD = standardized mean difference, SE = standard error, Total = number of participants, CI = confidence interval, IV = inverse variance, Random = random effect model, df = degrees of freedom.
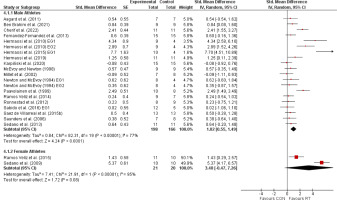
Figure 6
The effects of heavy loads resistance training (HL) compared with light loads resistance training (LL) on sport-specific performance in elite athletes.
Experimental = experimental group, Control = control group, SMD = standardized mean difference, SE = standard error, Total = number of participants, CI = confidence interval, IV = inverse variance, Random = random effect model, df = degrees of freedom.
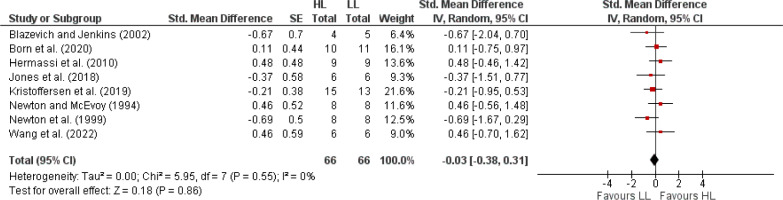
Discussion
The nature of each sport requires the development of specific skills to meet the demands and challenges of each sport, ensuring peak performance. Our analysis reveals that RT may play a pivotal role in sport-specific performance enhancement in elite athletes. The main conclusions of the conducted meta-analysis were that (i) RT produced large overall beneficial effects on sport-specific outcomes in elite athletes when compared with a control group of elite athletes, (ii) the effectiveness of RT in sport-specific performance was determined by a diverse range of factors, including the competitive level of athletes, the nature of outcomes, sex differences, and variations in RT methods, and (iii) there was a notable lack of randomized studies examining the influence of RT on sport-specific performance among international-level elite athletes, particularly those at Olympic or world champion levels, and a paucity of studies focusing on elite female athletes.
The findings of this systematic review revealed that RT, when used as a supplement to sport-specific training, offered large advantages (SMD greater than 1) for enhancing performance in various sports, from cross-country skiing (Losnegard et al., 2011), orienteering (Paavolainen et al., 1999) through handball (Hermassi et al., 2010) and soccer (Sedano et al., 2009) to water polo (Veliz et al., 2015). This enhancement demonstrates the versatile and impactful nature of RT in boosting sport-specific performance in elite athletes. A significant advantage was consistent across studies, as reflected by the high heterogeneity (I2 = 84%), suggesting that while RT is broadly effective, the degree of impact varies considerably among individuals. This variation could be due to differences in sport-specific demands, RT protocols, or individual athlete responses, underscoring the necessity for personalized RT regimens to optimize sport-specific performance enhancements in elite athletes. It is also possible that differences in prior experience with RT between athletes could have contributed to the variations noted above.
Interestingly, the subgroup analysis based on the level of competition revealed that RT had a more pronounced and significant effect on national elite athletes compared to their international counterparts. This may suggest that athletes at the national level likely have more room for physical improvement through RT compared to international athletes who may already be at or near their peak physical condition. Additionally, the observed low heterogeneity among international elite athletes might indicate more uniform training and performance standards at the highest sports level. In contrast, the diverse responses (high heterogeneity) within national elites could reflect a broader spectrum of training methods, physiological adaptations, and performance capacities. These insights point towards the need for a tailored approach in implementing RT, considering the athlete's current competitive level and specific needs (Loturco et al., 2023a). Thus, it becomes evident that a more specialized and advanced form of RT may be the key to unlocking further performance enhancements in international-level elite athletes. For instance, Zinke et al. (2018) found that in canoe sprint, where trunk muscles are crucial for both stabilizing the body in an unstable environment and generating propulsive forces, isokinetic training significantly enhanced athletic performance. Their study with world-class canoeists included a comprehensive 8-week isokinetic training program, emphasizing muscle hypertrophy and power. The findings revealed significant improvements in the peak torque of trunk rotators and established a strong correlation between the increased strength of concentric trunk rotators and peak paddle force, underscoring the efficacy of isokinetic training in boosting specific aspects of performance in elite canoe sprinters. Similarly, Schärer et al. (2019) explored the impact of a four-week eccentric-isokinetic training program on international and national top-level gymnasts, focusing on enhancing static strength elements in ring routines. This targeted approach yielded significant improvements in maximum strength for key elements such as the swallow and support scale. The success of this training regimen highlights the effectiveness of specific, tailored exercises for elite gymnasts. It is important to note that both the above-mentioned studies were single-group, non-randomized investigations. While they provided valuable insights into the effects of specialized training regimens on elite athletes, the lack of randomization and control groups in those studies limits the ability to generalize their findings. This highlights the need for more randomized controlled trials in this field to better understand the impacts of such training methods and to validate their effectiveness across a broader range of athletes. In addition, the smaller effect noted in international athletes does not necessarily suggest that RT was ineffective in that it is possible that cessation of current RT by such athletes could lead to a reduction in performance over time.
Typically, authors do not differentiate among various types of sport-specific outcomes. Both broad categories—such as running or swimming times—and more specific tasks like velocity in throwing or kicking are often analyzed together. To recognize this diversity and gain a more nuanced understanding of the impact of training interventions on sport-specific outcomes, we decided to separately examine the influence of RT on two distinct groups of outcomes: global and local. Global sport-specific outcomes, such as running, swimming, cycling, or rowing, engage multiple large muscle groups and often involve the entire body. These activities are typically considered whole-body movements and are used to assess overall performance in a given sport. Contrarily, local sport-specific outcomes focus on the performance of isolated muscle groups. This could be specific to the velocity of a kick in soccer, the accuracy of a throw in baseball or the power of a punch in boxing. They are crucial in sports where particular movements or actions are repetitive and critical for success. Meta-analyses of global and local sport-specific outcomes provide compelling evidence that RT has a differential impact on sport-specific performance, with a pronounced benefit for local sport tasks over global activities. On the one hand, this may reveal the multifaceted nature of global outcomes, indicating that they do not rely solely on one training method. On the other hand, it suggests that coaches have a tangible opportunity to effectively enhance technical elements of competitive tasks through RT. For instance, notable advancements in the velocity of ball-throwing or kicking have been observed in handball (Cherif et al., 2022; Hermassi et al., 2010; Hermassi et al., 2019), soccer (Ben Brahim et al., 2021; Campo et al., 2009) and water polo (Veliz et al., 2015). This evidence highlights the crucial need to understand how incorporating RT methods can specifically influence sport-related outcomes, thereby enabling a more effective and tailored approach to meet the distinct requirements of each sport.
An additional meta-analysis conducted as part of this study highlighted the impact of RT on sport-specific outcomes concerning sex differences. It is clear that RT benefits both male and female athletes, a finding that aligns with previous research (Kojić et al., 2021); however, the evidence suggests more substantial gains in male athletes. The observed positive trends in female athletes likely did not reach statistical significance, potentially due to the limited number of studies focused on elite female athletes (Campo et al., 2009; Veliz et al., 2015). This paucity of research on female athletes emphasizes the critical need for more comprehensive studies that investigate the effects of RT on sport-specific outcomes in elite female cohorts.
The findings of the final meta-analysis revealed no significant differences between heavy and light load RT, with notably low heterogeneity (I2 = 0%). These results imply that RT adaptations may align more closely with the unique performance requirements of their sports rather than the intensity of the load. This concept of specificity is particularly relevant in the context of team sports and multi-discipline events (Reilly et al., 2009), where performance requirements are diverse and require a holistic approach to training. Further, supporting the concept that RT adaptations are closely linked to sport-specific demands is the consistency observed across various RT studies in both individual and team sports used in this meta-analysis. This understanding may underscore the importance of a customized training approach, one that acknowledges and addresses the intricacies of each sport and athlete. It also highlights the need for training regimens that are not only physically demanding but also strategically aligned with the specific skills, tactics, and team dynamics essential for success in competitive sports.
The primary limitation of this study, which is also prevalent in RT literature, is the tendency to aggregate various types of sport-specific outcomes. This approach may obscure the distinct effects of RT on global versus local sport tasks, potentially masking the nuanced benefits of specific skills and highlighting the necessity for separate analyses to fully comprehend RT's impact on diverse performance metrics. This also highlights the need to identify sport-specific key performance indicators that are predictive of success, which can then serve as the outcome measures for future investigations. Future research should focus on the development of sport-specific resistance training protocols that account for the unique physiological and biomechanical demands of different sports, aiming to optimize individual athlete performance and reduce injury risk. Additionally, there is a need to explore the longitudinal effects of resistance training on athletes’ development, particularly how variations in training loads, frequency, and intensity over time influence sport-specific skills and performance outcomes. As noted earlier, there is a scarcity of research performed on women as compared to men looking into the magnitude of impact that resistance training has on sport performance. As such, future investigations should explore whether similar relationships exist between men and women and what are, if any, sex-specific differences. We also suggest that studies looking into potential thresholds of strength and power in relation to different levels of competition be performed. This may help solidify the understanding of how influential resistance training is for athletes at different performance levels, i.e., national versus international competition, and indicate potential implications of prioritization of resistance training in the overall training plan. Finally, the results of this review suggest that there exists a good deal of individuality in the magnitude of response to resistance training and the relevant relationship with sport performance. Future investigations may wish to explore this phenomenon in an effort to optimize outcomes for all athletes.
The systematic review and meta-analysis conducted herein underscore the significance of RT as supplementary to sport-specific training in enhancing performance of elite athletes. Resistance training has demonstrated large beneficial effects across a range of sports, indicating its efficacy in improving sport-specific performance, from endurance to high-power sports and from individual to team sports. However, the influence of RT is not uniform; it varies according to the athlete's competitive level, sex, and the specific demands of their sport. Notably, the impact of RT is more pronounced in national elite athletes than their international counterparts, suggesting that the latter may require more advanced and individualized training interventions (Loturco et al., 2024). Further meta-analysis revealed that RT had a more significant impact on local sports tasks compared to global activities, indicating its differential influence on sport-specific performance. The studies also identified a critical research gap regarding the effects of RT on female elite athletes, emphasizing an urgent need for further investigation. Overall, the findings advocate for the principle of specificity in RT programs, with a balanced integration of heavy and light loads tailored to the athlete's unique needs and the performance requirements of their sport. Finally, this review calls for more randomized controlled trials, especially among international-level elite and female athletes, to confirm the generalizability of these findings and to refine RT protocols for optimal sport-specific outcome enhancement.
Conclusions
This study underscores the importance for coaches to design RT programs that are specifically tailored to the unique needs of each athlete, factoring in their sport, competitive level, and sex, to maximize sport-specific performance enhancements. The current body of literature suggests that at lower levels of competition, resistance training provides greater impact on sport performance, perhaps due to differing levels of conditioning at the international level. It also highlights the necessity of developing advanced and individualized RT interventions for international elite athletes and addressing the research gap between the sexes, ensuring that training protocols are optimized for all athletes.