Introduction
Metabolic acidosis is a temporary consequence of an intense burst of exercise, such as a sprint by runners or cyclists, which limits an athlete's performance (Bangsbo et al., 1993; Robergs et al., 2004). The body's first line of defence against such change in internal pH is provided by buffer systems (protein, phosphate, and bicarbonate) when blood accepts hydrogen ions from the lactic acid produced in muscle tissue, which is one of the major end products of anaerobic metabolism (Nelson and Cox, 2017). A shred of sufficient evidence indicates that alkaline agents can modify the blood buffering system by increasing the amount of bicarbonate concentration in the blood (increased blood pH), which in turn allows for a greater accumulation of lactate in the muscles during intense exercise and, thus leads to improved anaerobic performance (Sahlin, 1978; Zajac et al., 2009). For this reason, one of the recent interest in sports science has been the drinking of alkaline water (AW), which has been shown to enhance athletes' anaerobic performance, hydration status and increase both fasting and post-exercise arterial blood pH (Chycki et al., 2017, 2018; Kurylas et al., 2017; Ostojic and Stojanovic, 2014; Roczniok et al., 2013). However, the exact alkalizing mechanism in terms of lactic acid accumulation and utilization after exercise is still unclear. Chycki et al. (2017, 2018) have shown that after drinking AW for 2-3 weeks, blood lactate levels at rest significantly decreased, but increased post-exercise, suggesting better lactate utilization.
Lactic acid has long been thought to be the culprit of muscle fatigue and pain as the anaerobic breakdown of glycogen leads to its intracellular accumulation. However, recent evidence on mammalian muscle has shown that reduced pH may have little influence on muscle contraction, and it is most likely the inorganic phosphate which is the major cause of muscular fatigue (Posterino et al., 2001; Westerblad, 2002, 2016). In the same way, there are currently contradictory opinions (Coco et al., 2009, 2016, 2020; Duncan et al., 2017; Mroczek et al., 2011; Tanaka et al., 2018; Tsukamoto et al., 2016) on how high blood lactate levels (induced either by exhaustive exercise or by an intravenous infusion of a lactate solution) may affect various psychomotor processes, especially simple reaction time.
Simple reaction time demands intense, simple attention and is considered an essential ability for many sport disciplines, especially the ones concerning visual stimulus (Cojocariu, 2011). For example, Perciavalle et al. (2014, 2016) have found that attentive capabilities and performance after an exhaustive exercise worsened, most likely due to increased blood lactate levels. Coco et al. (2020) also found increased reaction time and blood lactate after intense exercise, which were strongly correlated. On the other hand, Mroczek et al. (2011) found an initial decrease in reaction time despite increased blood lactate during and after volleyball sets.
Therefore, based on all the studies mentioned above, reporting both, improved anaerobic performance (linked to better lactate utilization and hydration status) after drinking AW and the effects of high blood lactate levels on reaction time, we investigated the effects of high mineral AW consumed over three consecutive days on reaction time after anaerobic exercise, which is a novelty of this study.
Methods
Participants
Twelve young male students of the Faculty of Physical Education and Sport, Charles University, with an average age of 21.1 ± 1.3, were recruited for the study. The inclusion criteria comprised increased anaerobic performance and cognition at a competitive level (e.g. a sub-elite competitive athlete), age between 18 – 25 years and current training of at least three times a week. All participants were classified as healthy and had a valid sports medical examination, which allowed them to participate in the experiment. They did not take any medications or any performanceenhancing supplements for at least four weeks before the study commenced (exclusion criterion). All participants signed informed consent after being informed about the study, including the possible risks. The study was conducted with the consent of the ethics committee of the Faculty of Physical Education and Sport, Charles University, Prague, Czech Republic, conforming to the ethical standards set by the Declaration of Helsinki (World Medical Association, 2013). Participants' characteristics are presented in Table 1.
Study Design
A pilot study with a double-blind, randomized, placebo-controlled crossover design was applied (Figure 1). Participants were randomly divided using free online statistical computing web programming (www.graphpad.com/quickcalcs) that generated the randomization schedule into two groups (n = 6) with different conditions: the consumption of 1) AW or 2) regular table water (RTW). At first all participants performed the baseline reaction time tests. A wash-out period of two weeks was applied to prevent a carry-over phenomenon before crossing-over. Participants were instructed to ingest 2.5 - 3 L (based on their body weight; i.e. at least 35 ml∙kg-1 of body weight a day) of either AW or RTB (blinded) a day for three consecutive days (Kurylas et al., 2018). The water for the three-day consumption was provided in 6 x 1.5 L bottles; two bottles were to be consumed each day throughout the 24 hours (e.g. in the morning after awakening, during the day, and before going to sleep). Before physical testing, lactate concentration was evaluated from capillary blood, and urine specific gravity was measured as well. The reaction time for visual and auditory signals was evaluated immediately after each physical test, along with the Rate of Perceived Exertion (RPE). The recovery time between the two physical tests was approximately 3 minutes, which corresponded to the execution time of the speed of reaction test. After finishing the second physical test, the urine analysis was performed again. The capillary blood was retaken in the fifth minute after the second step-test for evaluation of lactate concentration. To ensure similar conditions in terms of sports activity, sleeping time, and diet, participants were instructed to (1) omit strenuous physical activity the day before the examination; (2) three days before the testing itself, drink only the given amount of water (alkaline water or regular table water); (3) obey the requirement of 8 hours of sleep a day; (4) refrain from consuming alcohol, dietary supplements, and caffeinated products, and (5) follow an isocaloric mixed diet (55% carbohydrates, 20% protein, and 25% fat). Each trial was performed at the same time of the day to avoid circadian variations. The study lasted for a total of 3 weeks.
Alkaline Water
Commercially available glacial water with a pH of 8.8 was used in the study. The water was relatively highly alkaline compared to other commercially available products with high dissolved oxygen content (~ 8.5 mg∙l-1) and low mineralization (~ 59 mg∙l-1). It contained Na+ 9.52 mg/l; Ca2+ 4.47 mg∙l-1; Mg2+ 1.66 mg∙l-1; Al+ 0.0193 mg∙l-1, and Cl- 8.18 mg∙l-1, other ions were present in small amounts. The total hardness of water was 0.180 mmol.l-1. Odour and taste were indistinguishable from the RTW.
Physical Test
A modified version of the high-intensity step-test was used in the study (Perciavalle et al., 2016). In this study, we used a 50 cm high step, and we set the execution time to 2 minutes. Ascents and descents were performed alternately with the right and the left leg, where one leg always remained on the podium, and the tested person was not allowed to rest hands on thighs. An authorized member of the research staff supervised the correct technical execution of the test, tracked the time, motivated the participants, and recorded the results. The performance in watts (W) was calculated as follow:
where t = test duration [s]; F = force [N]; s = distance [m]; m = body weight [kg]; h = step height [m]; c = number of steps; 1.33 = coefficient for positive (1.0) and negative work (0.33). Reaction Time
Special software developed for this purpose at the Department of Sports Diagnostics, at the Faculty of Physical Education and Sport Charles University, was used to measure reaction time. Briefly, the tested person had to press a button connected to the computer when a visual (on the screen of the computer) or an auditory signal (beep) appeared. The tested person had to first react as soon as possible to twenty visual and then to twenty acoustic signals for 80 s. The five fastest and five slowest reaction times were automatically deleted from the average reaction time calculation. To avoid settling habituation, signals were randomized with intervals spanning between 1 and 3 s. The reaction time in [s] was measured for visual and auditory signals separately.
Blood and Urine Tests
20 μl of capillary blood was collected from the fingertip to determine lactate concentration before and after exercise. Blood samples were analysed by the analyser SUPER GL - Biovendor. Urine specific gravity before and after exercise was analysed using the TIPA LH-T50 Refractometer.
Rate of Perceived Exertion
The 10 point Rate of Perceived Exertion (RPE) scale was used in this study (Borg, 1982). The RPE is a self-reported scale ranging from 1 = extremely easy to 10 = extremely hard exercise, and the participants were familiarized with the scale before the start of the experiment.
Data Analysis
Our internal reliability data measured by the intra-class correlation coefficient (ICC) for both exercise and reaction time protocols indicated an excellent level of reliability for all the considered variables (ICC ranging from 0.96-0.99). Means and standard deviations (SDs) were calculated for each variable. Because of the small sample size, we used non-parametric statistical methods for repeated measures (Sullivan et al., 2016; Yan et al., 2017). The Friedman test (α = 0.05) with the Wilcoxon signed ranks test with Bonferroni correction in the posthoc analysis were used to test our hypotheses. In the Bonferroni correction, the standard level of significance was divided by the number of comparisons α = 0.05/6 = 0.008; therefore, the statistically significant result after the correction was when p < 0.008. All the statistics were carried out in the statistical software IBM SPSS Statistics 24. Graphs for the reaction time were created separately in MS Excel.
Results
There were no significant changes observed in reaction time for both visual and auditory signals after drinking AW or RTW when compared to the resting values. Graphical representation of both reaction times is provided in Figure 2.
Figure 2
Reaction time changes to visual )p = 0.685( and auditory signals )p = 0.249(after ingesting alkaline water.
Note. AW - alkaline water; RTW - regular table water.
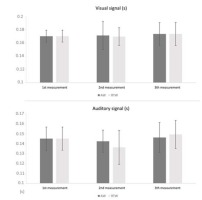
Performance measured in watts (W) in the first (853.2 ± 128.7 vs. 739.6 ± 107.6) and second (814.4 ± 127.6 vs. 688.6 ± 86.1) step-tests was significantly higher after the intake of AW when compared to RTW, respectively. Performance in the second step-test was not significantly different from the first step-test after both AW and RTW.
There was no significant difference in the RPE between both groups after both tests. The RPE significantly increased (p = 0.004) after the second step-test (when considering the first step-test values) in RTW. There was a significant difference between the first measurement in AW and the second measurement in RTW.
No significant difference was observed between the initial lactate concentration after AW and after RTW. There was a significant increase in lactate concentration after the step-tests under both conditions.
No significant difference was observed in urine specific gravity.
Graphical representation of performance in step-tests, RPE, lactate concentration and urine specific gravity is provided in Figure 3.
Discussion
In this study, we investigated the effects of high mineral alkaline water consumed over three consecutive days on reaction time after anaerobic exercise. This study found no effect on reaction time for both visual and auditory signals in healthy young males. However, a significant difference in anaerobic performance (measured by a step-test) was observed.
Recent studies have found a positive effect of alkaline water ingestion on anaerobic performance, which stems from improving the acid-base balance, lactate utilization and hydration (Chycki et al., 2017, 2018; Kurylas et al., 2017). We, therefore, hypothesized that it might also affect attentive performance, such as simple reaction time, since several recent studies have linked high blood lactate levels to various psychomotor processes both positively and negatively (Coco et al., 2009, 2016, 2020; Mroczek et al., 2011; Perciavalle et al., 2014).
In our study, reaction time did not change when compared to pre-exercise values, which can be considered positive, since athletes with high levels of blood lactate even at rest, had attentional performances, including reaction time-limited (Coco et al., 2009). In terms of the reaction time and lactate concentration, the results of our study partially support the findings of Mroczek et al. (2011) who measured reaction time at rest and blood lactate concentration pre-game and during four volleyball sets and found that reaction time decreased during the first set significantly when compared to the pre-game test and then remained at the same level at all time. In their study, the association between reaction time and lactate was not calculated, due to the study's nature. However, future and more extensive studies conducted preferably on different populations are needed to investigate this phenomenon further.
Generally, our study results are more likely to be attributed to the good hydration status in both groups. Good hydration can have a significant positive influence on aerobic and anaerobic performance, including people's mental state. It has been shown that even a minor 1% hypohydration influences a variety of cognitive functions (Karpęcka and Frączek, 2020), specifically the speed of reaction (Cian et al., 2001; Tomporowski et al., 2007). Indeed, reaction time is controlled by the central nervous system and is affected by many factors, including age, gender, stimulus (type and intensity), arousal, fatigue and exercise (Cote et al., 2009; Der and Deary, 2006; Kashihara and Nakahara, 2005). For example, Tsukamoto et al. (2016) showed an improved executive function, including reaction time after high-to-moderate intensity interval exercise, although Perciavalle et al. (2014, 2016) and Coco et al. (2016; 2020) found the opposite. Nevertheless, in our study, we intended to investigate only the differences in reaction time after the three-day ingestion of alkaline water vs. tap water to provide pilot data to support future studies.
In our study, we found significantly higher performance in the two step-tests after the threeday ingestion of alkaline water. In terms of lactate concentration (measured by capillary blood), our initial levels did not differ significantly between the two groups; even though an increased lactate level is noted following the ingestion of alkaline water, which is in partial agreement with Chycki et al. (2018) and Kurylas et al. (2018). The muscle pH of trained athletes can drop from a resting value of 7.0 down to 6.4 following intense exercise, and bicarbonate-CO2 accounts for more than 90% of the plasma buffering capacity (Robergs et al., 2004). In our study, we did not find any significant increments in urine specific gravity, unlike in the two previous studies (Chycki et al., 2017; Kurylas et al., 2018), which was something expected (three consecutive days vs. six consecutive weeks (Kurylas et al., 2018)). Our results have instead served to check the hydration status of both groups, which was nearly identical, suggesting good participant's compliance, and as mentioned above, it may be the main reason why reaction time was unchanged in both groups.
Nevertheless, the six-week study (Kurylas et al., 2018) showed that highly alkalized water delayed muscular acidosis during anaerobic exercise, acted as prevention of dehydration, and improved recovery. As the RPE scale can 'indirectly' assess all physiological signs (the sensation of fatigue and pain after such exercise) of redox homeostatic mechanisms (Borg, 1982), it appears that this greater muscle buffering capacity and enhanced removal of protons, resulting in increased glycolytic ATP production, may be responsible for the improved anaerobic performance following alkaline water ingestion. Such an effect of alkaline water ingestion before anaerobic exercise is similar to sodium or potassium bicarbonate supplementation. The use of sodium bicarbonate as an alkaline agent can be dated back to 1928 when Haldane and his physiologist colleague, Davies (Haldane, 1928), decided to experiment on themselves how the body controls blood pH. Since then, the ingestion of sodium bicarbonate has proven effective in speed endurance sports; however, its use has been limited as it often causes gastrointestinal distress or metabolic alkalosis due to sodium overload (McNaughton et al., 2008). These supplements have the potential to modify the adaptive response to endurance training by affecting acid-base balance, redox status, reactive oxygen species (ROS) signalling, or cumulative training load, thereby affecting the cellular signalling responses to training (Rothschild and Bishop, 2020). Therefore, the idea of increasing the capacity of the body's buffering system by ingesting alkaline agents to enhance anaerobic performance stems from the ionic biochemical interplay (Chycki et al., 2018; Coco et al., 2016). Although there is a large body of evidence to support the use of sodium bicarbonate for sports performance, its use has been associated with possible gastrointestinal side effects (Kahle et al., 2013). Contrary to this, it seems that alkaline water can serve as an effective alternative to sodium bicarbonate without the mentioned side effects, improving an athlete's anaerobic performance (Chycki et al., 2017, 2018; Kurylas et al., 2018; Ostojic and Stojanovic, 2014). Future studies could consider comparing the use of sodium bicarbonate, alkaline water, as well as an isotonic beverage to assess not only the negative side effects, but also anaerobic performance following these three supplements.
It should be noted that some methodological considerations are involved in this study. First, the main limitation of this study is the small sample size due to the pilot nature of the investigation. For this reason, we used nonparametric statistical testing as the recommended approach in medical and biomedical research (Sullivan et al., 2016; Yan et al., 2017), along with the calculation of effect sizes to account for this problem. Another limitation is that this study did not measure the acid-base balance, which, otherwise, could provide a more detailed mechanistic insight into our findings. Future studies should ideally focus on measuring this variable alongside and comparison to other food supplements like hydrogen-rich water (Timón et al., 2021) or bicarbonates (Chycki et al., 2021). In our study, we used natural AW with pH 8.8, which is not the same as extremely high AW with pH 10, for which negative health consequences in rats have been reported (Merne et al., 2001; Watanabe et al., 1997).
Conclusions
In conclusion, the results of the present study indicate that ingestion of high mineral alkaline water for short, three-day duration has no effect on reaction time. However, alkaline water seems to positively affect anaerobic performance, which needs to be further confirmed by future studies with a larger number of subjects.