Introduction
The non-medical use of Anabolic Androgenic Steroids (AAS) has been considered a widespread public health problem in recent decades (Leifman et al., 2011) due to the dramatic increase in the prevalence rate of its use, not only among elite athletes, but also among “recreational” exercise practitioners (Kanayama et al., 2018). A meta-regression of 187 studies stated that the overall prevalence rate was 21.7% in the Middle East, 4.8% in South America, 3.8% in Europe, 3.0% in North America, 2.6% in Oceania, 2.0% in Africa, and 0.2% in Asia, significantly greater among men, adolescents, and recreational athletes (Sagoe et al., 2014).
Chronic and supraphysiological use of AAS can promote side effects including infertility (Christou et al., 2017) and gynecomastia (Rahnema et al., 2015) in men, and virilization and alteration in the libido in women (Rocha et al., 2014). In addition, it is associated with liver injury (Schwingel et al., 2011), sudden death (Frati et al., 2015; Nieschlag and Vorona, 2015), increased aggressiveness (Maior et al., 2013), and even death from urban violence due to increased aggressiveness (Choi and Pope, 1994). The indiscriminate use of AAS can also lead to several cardiometabolic disorders, such as dyslipidemia, infarction, stroke, inhibition of angiogenesis, systemic inflammation, and vascular dysfunction (Santos et al., 2014; Vanberg and Atar, 2010). These effects explain the increase in blood pressure to hypertensive levels, which is regarded as another notorious AAS side effect (Rodrigues-Junior et al., 2017).
Furthermore, the impairment of the response to post-exercise hypotension as a new side effect of the use of AAS has recently been demonstrated (Rodrigues-Junior et al., 2017). In the aforementioned study, AAS users did not reduce blood pressure after an aerobic exercise session. However, the possible associated mechanisms were not presented. There is also evidence that AAS promote a reduction in the parasympathetic resting activity in animals (Pereira-Junior et al., 2006) and humans (Vanderlei et al., 2009). Hence, we raised the hypothesis that users of AAS may present dysfunction of autonomic nervous activity after an aerobic exercise session. Thus, the present study was designed to investigate the acute effect of aerobic exercise on heart rate variability (HRV) among bodybuilders using AAS.
Methods
Participants
Nine AAS male users (21.8 ± 2.8 years old) and eleven male controls (22.1 ± 4.5 years old) were recruited for this study. They performed strength exercises for bodybuilding competition at the professional level. Volunteers trained at least six times a week and had been practicing bodybuilding for at least two years. None of the participants presented any chronic degenerative disease, nor did any of them use anti-hypertension medicines or nutritional supplements. No smokers or alcohol consumers were included as volunteers for this study. Participants who refused or presented difficulties performing the exercise session were excluded from the study.
The AAS group had been using AAS at baseline for at least 2 years, ranging from 2 to 6 complete cycles. The average weekly AAS dosage was 300 ± 161.9 mg. The most widespread AAS used by participants were testosterone propionate (33.3%), testosterone propionate + stanozolol (11.1%), testosterone propionate + oxandrolone (22.2%), dianabol (22.2%) and testosterone enanthate + drostanolone propionate + boldenone (11.1%).
Experimental Protocol
All volunteers were anthropometrically evaluated and instructed to refrain from exercise for 48 h before the experiment test trial, as well as to avoid the intake of supplements and food with stimulants, such as coffee, tea, soda, energy drinks, and chocolates. HRV measurements were performed at rest, immediately after the exercise session, and in the recovery period every 10 min until completing the 60 min recovery period. The entire protocol is shown in Figure 1.
Figure 1
Schematic view of the exercise and evaluation protocol. HR, heart rate; HRV, heart rate variability.
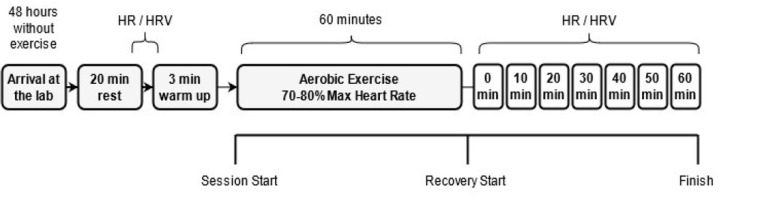
The exercise protocol was performed between 2 and 4 PM. One aerobic exercise session was performed on a treadmill (ProAction BH fitness, Puerto del Carmen, Spain). Firstly, volunteers began with a warm-up run on the treadmill for 3 min at self-chosen intensity, considered mild by the volunteers (<60% of HRmax). Immediately after the warm up, they gradually increased the speed by 1% every minute until the prescribed heart rate (HR) was reached (between 70 and 80% of HRmax). Once the HR was within the prescribed zone, the treadmill speed remained unchanged until the end of 60 min of exercise, according to recommendations for this population (American College of Sports Medicine, 2002).
The HR was measured with a V800 heart rate monitor (Polar® Electro Oy, Kempele, Finland), previously validated to record beat-to-beat heart rate and heart rate variability analysis by Giles et al. (2016). For pre- and post-exercise measures, participants remained seated in a quiet environment with temperature between 24 and 27⍛C. Autonomic activity was determined by recording the variability of the R-R heart rate intervals under resting conditions 5 min before exercise, immediately after exercise and in the recovery period at the 10th, 20th, 30th, 40th, 50th and 60th min. Before each evaluation, there was a 5-min period of R-R interval stabilization (except for measurement immediately after exercise). The subsequent five minutes were extracted from the monitor and converted into text using Polar ProTrainer software. The initial and final records were discarded, thus only 300 heart beats were analyzed by HRV analysis software.
HRV was measured according to linear methods (time and frequency). Time domain measures included RR (interval means), SDNN (standard deviation of the intervals) and RMSSD (square root of the mean squared difference between adjacent RR intervals), expressed in ms. For the frequency domain, analysis included the LF/HF - ratio spectral components of low frequency (LF) and high frequency (HF). They were calculated in order to indirectly reflect a shift to sympathetic or parasympathetic predominance. The HF component acts in HRV reflecting parasympathetic modulation, whereas the LF component includes sympathetic and parasympathetic function.
Statistical analysis
The data are presented as mean ± standard deviation of the mean. The normality and homogeneity of the data were analyzed via Shapiro-Wilk and Levene’s tests, respectively. Since HRV did not present normal distribution, natural logarithmic (ln) transformation was employed. An independent Student’s t-test was used to compare differences between initial group characteristics. Repeated measures ANOVA was used to compare pre- and post-exercise HRV recovery. Cohen’s d effect size was used to calculate the magnitude of the difference. Values of 0–0.19, 0.20–0.49, 0.50–0.79, and >0.80 were considered trivial, small, moderate and large, respectively (Cohen, 1988). The level of significance was established at p < 0.05. The entire statistical analysis was performed using the SPSS version 20.0 statistical software (SPSS, Inc., Chicago, IL, USA).
Results
Data regarding resting characteristics are presented in Table 1. Participants were statistically similar in age, body mass, body height, and VO2max, differing only in body mass index (BMI) (p = 0.02). At rest, the AAS group had lower diastolic blood pressure (p = 0.01) and similar systolic blood pressure, mean arterial pressure, and HR. The AAS group presented lower initial values for the standard deviation of the interval (SDNN) (p = 0.04) variables and higher initial value of the LF/HF (p = 0.03) than the control group.
Table 1
Initial values of body composition, blood pressure and heart rate variability of the study participants (n=20).
[i] Data are: BMI, body mass index; SBP, systolic blood pressure; DBP, diastolic blood pressure; MBP, mean blood pressure; VO2max, maximum oxygen uptake; RHR, resting heart rate; RR, interval means; SDNN, standard deviation of the intervals; RMSSD, root mean square successive difference of the RR intervals; LF, low frequency spectrum; HF, high frequency spectrum; LF/HF, ratio of spectral components of low frequency (LF) and high frequency (HF) .* Significant difference Control vs. AAS (p < 0.05).
Figure 2 shows HR, RMSSD, SDNN and LH/HF values before, after and during the recovery. The aerobic session caused an increase in the HR with recovery in the post-exercise period. However, AAS users presented a consistently higher HR than the control group at all times at 0, 30, 50, and 60 min after exercise (Figure 2, Panel A).
Figure 2
Heart rate (panel A), heart rate variability in time (RMSSD, panel B), Standard Deviation of the intervals (SDNN, panel C) and frequency (LF/HF, panel D) domains at pre-, post- and during 60-min of recovery after the aerobic exercise session. AAS, anabolic androgenic steroids users; RMSSD, square root of the mean squared differences of successive RR intervals; LF/HF, low-frequency/high-frequency ratio (sympathovagal balance). Data are presented as mean ± standard deviation of the means; * Significant difference Control (n=11) vs. AAS (n=9); # significant intragroup differences at rest; p < 0.05.
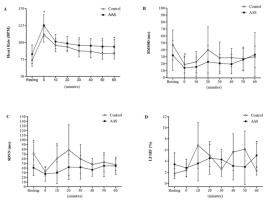
The aerobic session significantly reduced the RMSSD both in the AAS (31.1 ± 21.7 to 14.0 ± 15.8 ms; p = 0.005, d = 0.9) and in the control group (46.9 ± 21.5 to 18.9 ± 12.1 ms; p = 0.002, d = 1.6) compared to before exercise (Figure 2, Panel B). The response to exercise and recovery was similar in both groups, despite the descriptively lower values for AAS users in all measurements performed (Figure 2, Panel B). As for the SDNN (Figure 2, Panel C) variable, there were significant differences between the experimental and control groups before and in the 10th min of post-exercise recovery.
The LF/HF ratio did not change immediately after the cessation of exercise considering rest values in both groups. However, during the recovery period, this balance underwent a slight and gradual increase in the AAS group up to 40 min and remained at these values until the end of the recovery session. As a result, the values were significantly higher than those of the control group at the 30th and 60th min after exercise.
Discussion
This study provides evidence of differences in nervous autonomic control at rest and immediately after aerobic exercise in AAS users, but a similar recovery for both AAS and control groups. As far as we know, only few studies have investigated the behavior of the post-exercise autonomic system in AAS users. Resting autonomic dysfunction has already been reported in studies with animal and human models. Studies using animal models found significant reductions in parasympathetic activity and increased cardiac sympathetic modulation after chronic administration of high doses of AAS (Marocolo et al., 2013; Pereira-Junior et al., 2006). This behavior was also observed in two studies carried out with humans using supraphysiological doses (Maior et al., 2013; Neto et al., 2017).
Although there was no significant difference in the HR at rest, we observed that individuals in the AAS group had higher HR values (12.3%) compared to those of the control group (82.8 vs. 73.7 BPM, respectively). That has significant clinical relevance since a relationship between HR values and acute myocardial infarction has been reported (Berton, 2002), in addition to a greater chance of atherosclerotic plaque rupture in the coronary artery in men with HR> 80 BPM (Heidland and Strauer, 2001). After exercise, the HR showed a quick (although incomplete) decline in the first minutes of recovery with eventual return to pre-exercise levels, with greater reduction in the control group, corroborating with other studies (Al Haddad et al., 2011; Stanley et al., 2013).
Likewise, the AAS group presented lower HRV (13.9%) at rest (709.9 ± 160) when compared to the control group (808.0 ± 107). Previous studies have already reported autonomic dysfunction at rest, both in animal models and humans. Maior et al. (2013) showed that R-R interval levels at rest were lower and the LF/HF ratio was higher in the AAS group compared to control subjects. This is probably due to an increase in the sympathetic activity and a decrease in the parasympathetic activity. Despite the fact that the previous study differed from ours regarding the aerobic exercise test adopted (cycle ergometer), time of AAS administration (5 vs. 2 years), and the dosage per week (410 ± 78.6 vs. 300 ± 161.9 mg), their data are similar to ours regarding RMSSD and the LF/HF.
AAS users have lower HRV and cardiac remodeling at rest compared to non-users (Seara et al., 2019), which lead to a higher cardiovascular risk and sudden cardiac death (Hoshi et al., 2021).
For the SDNN variable, the AAS group showed significantly lower values (42.9%) than the control group (p = 0.04). Studies indicate that SDNN values below 70 ms present an increased risk of developing cardiovascular diseases (La Rovere et al., 1998). Individuals with SDNN <50 ms, as observed in our study, are 5.3 times more likely to die (Hoshi et al., 2021; Kleiger et al., 1987). To the best of our knowledge, this is the first study to evaluate HRV in bodybuilders during a 60-min post aerobic exercise recovery period. A similar study using 22 recreational athletes observed lower values before and after exercise for the RR, RMSSD, LF and HF variables in AAS users (Maior et al., 2013). However, they assessed HRV only 5 minutes post-exercise.
Figure 2 shows tachograms representative of the AAS and control groups, at rest and during 60 min of recovery. The control group showed a greater reduction in SDNN (73.4%) in the first 10 min of recovery compared to the AAS group (52.4%). However, the control group returned to pre-exercise values more quickly, in the first 20 min of recovery. During recovery, SDNN of the AAS group remained similar to that of the control group, which shows that the reduction in parasympathetic activity can occur during and at the end of exercise in AAS users, but returns to the resting state after 20 min of recovery regardless of the use of AAS.
HRV analysis has been widely used to evaluate autonomic function in several sports disciplines (Picabea et al., 2021; Ravé et al., 2020). During exercise, there is an increase in sympathetic modulation and a reduction in vagal modulation. However, soon after exercise, a return to pre-exercise values is expected. The average value of the RMSSD index in the AAS group after exercise was higher (by 22.2%) than that of the control group (26.34 and 20.49, respectively). In addition, the control group showed total recovery of the vagal index (RMSSD) in the first 20 min of recovery, while the AAS group recovered completely only 60 min after the start of the recovery.
The LF component, related to both vagal modulation and sympathetic modulation, was lower (by 13.5%) in the AAS (485 ms2), when compared to the control group (561 ms2). This is an indicator of sympathetic hyperactivity, since sympathetic activity contributes to by approximately 15% more than vagal activity for this index (Piccirillo et al., 2009). In addition, this lower LF power may reflect a lower parasympathetic HF activity, which was by 45.5% lower in AAS users.
Parasympathetic control, measured by HF power, was lower among users of AAS, apparently as a result of reduced vagal reactivation (delay in parasympathetic control), as previously described in other studies (Cole et al., 1999; Santos et al., 2014). In addition, AAS users had an LF/HF ratio higher than that of the control group (by 47.1%), which is an indicator of an increased risk of cardiovascular events, corroborating other authors (Santos et al., 2014).
Since vagal imbalance is associated with increased blood glucose and degenerative chronic diseases, an increase in the sympathetic activity can affect several physiological responses (Maior et al., 2013; Neto et al., 2017). In fact, this study is an extension of research in which we demonstrated impaired systolic post-exercise hypotension (PEH) as a new adverse effect of AAS usage (Rodrigues Junior et al., 2017).
Sympathetic activity is one of the main mechanisms to promote the PEH, which is impaired by the usage of AAS, as we previously reported (Rodrigues Junior et al., 2017). In the present study, we demonstrated that the LF/HF balance can impair PEH in AAS users. Previous research indicates that the increase in parasympathetic activity and endothelial-mediated dilation after exercise play an important role in PEH (Halliwill, 2001). Therefore, we can assume that lower parasympathetic activity and the absence of PEH occur concomitantly in AAS users.
In conclusion, this study verified differences in cardiac autonomic responses at rest and after aerobic exercise between AAS users and controls. AAS users had autonomic dysfunction and recovered less efficiently, but only at the end of the exercise. Further research is recommended to verify whether sympathetic hyperactivity or parasympathetic hypoactivity is the cause of these adverse effects of the use of AAS.