Introduction
As in other gymnastics disciplines or sports such as synchronized swimming or figure skating, the determination of sporting performance in artistic gymnastics relies on the judge’s estimation concerning the movement quality of the presented skills (Plessner and Haar, 2006). Therefore, perceiving and evaluating skills play a crucial role for judges, coaches, and the athletes themselves. While judges allocate points in a competition to identify the best athlete, coaches and athletes try to find sources of error to improve the movement. For both sides, the main idea is to determine the movement quality of a particular skill or routine and to discriminate between bad and good movement quality as precisely as possible (Mercier and Heiniger, 2018). In gymnastics, performance and thereby movement quality-determining aspects (i.e., straight body), by which the skills should be evaluated, are described in the code of points (Arkaev and Suchilin, 2004; FIG, 2017). To obtain a performance score, the presented skill has to be compared with an optimal model (Ste-Marie, 1999) which is characterized by certain kinematics.
It is suggested that movements are organized and stored in memory as a cognitive representation. These cognitive movement representations are based on the temporal and spatial kinematic features of movements acquired during training (Schack and Ritter, 2009) and vary by expertise. According to Schack’s architecture model (Schack, 2004; Schack and Ritter, 2009), mental representations consist of functional and sensory features. Functional features refer to movement goals utilizing the anticipated effects of the movement. The sensory features that were integrated into the movement through cognitive chunking refer to the perceptual effects of the movement. There is an increasing amount of research addressing the mental representation of movements in terms of their functional states and sensory events, as well as the presence of kinematic information (Schack, 2003). In sports such as artistic gymnastics or dancing, not only are the anticipated effects of a movement of importance, but also it is essential to evaluate the movement quality of a skill as a whole. From that point of view, not only the representation of the detailed action steps in a row is relevant, but also the representation of the relationship of kinematic variables over the whole time and space the movement is performed. To evaluate a gymnastics skill, all biomechanical information has to be summed up and integrated into an evaluation.
It is assumed that when perceiving movement, kinematic information, which is distributed over the whole time and space, is taken into account (Troje, 2002). The perceptual process may be moderated by aspects such as observers’ expectations or expertise. Expertise differences could be found concerning the perception of biological motion in daily life, where the movement recognition seems to be influenced by the observers’ familiarity with the observed action (Aglioti et al., 2008; Loula et al., 2005) and by the perception of different complex dance movements (Bläsing and Schack, 2012; Calvo-Merino et al., 2010).
Due to the results of research concerning motor-visual expertise and the underlying theoretical framework of the common coding theory (for a broader view of this model, view Prinz, 1997; Hommel et al., 2001), it could be assumed that in gymnastics, there may be differences between not only experts and novices, but also visual and motor experts concerning the perception and information processing of sports skills (Kim et al., 2019). By means of the specific and comprehensive qualifications regarding the quality-determining criteria, coaches and judges should gain an exact knowledge about the different skill executions in terms of their kinematic features and their matched quality scores. Therefore, it could be assumed that visual experts, such as coaches or judges who have a more detailed and precise representation of this optimal execution model, are consequently better to observe movement errors than motor experts (athletes) or novices and might determine a more accurate estimation of the movement quality (Land et al., 2013; Ste-Marie, 2001).
The question remains whether movements with a more similar structure of biomechanical variables were seen as more similar to movements with a less similar structure and whether a similar structure of movement variables leads to a similar judgment of movement quality. While there is research dealing with searching for the path between biomechanical aspects of movement and the functional elements of movement organization, there is a missing link between biomechanics and the perceived movement quality. In sports, where the judgment relies on subjective perceptual analysis, the relationship between the biomechanics and the perceived movement quality is poorly understood.
The aim of this study was to address the perception and evaluation of movement quality. Therefore, two research questions were investigated. The first was to identify the relationship between the kinematic structure of complex gymnastics skills and the evaluation of the movement quality. The second aim was to identify the influence of motor and visual expertise on the mental representation of the movement quality of complex gymnastics skills. This investigation was performed using a cluster analysis and its resulting tree diagram as well as a correlation analysis. If the judgment of the movement quality of gymnastics skills is based on the holistic kinematic pattern of the skills, the tree diagram of the pattern of the perceived movement quality, and the tree diagram of the kinematic pattern of different performances of the skill should show a similar pattern. If the pattern of the perceived movement quality is similar between gymnastics motor experts, gymnastics visual experts, and novices, the resulting tree diagrams should show a similar pattern.
Methods
Participants
In total, N = 30 participants with different levels of gymnastics expertise were recruited for the study. The number of participants was derived from a power analysis when expecting a medium effect (Cohen’s f > 0.25, type I error probability 5%, type II error probability 20%) and is comparable to similar studies conducted by Lander and Lange (1992, 1996) as well as Schack and Mechsner (2006). The group of motor experts consisted of n = 10 participants who were active in gymnastics as athletes for a minimum of three years and were still active at the time of investigation (age: 18.80 ± 7.28). The group of visual experts included n = 10 participants who were active in gymnastics as judges or coaches for a minimum of three years and were still active at the time of investigation (age: 46.90 ± 17.57). The group of novices included n = 10 participants who were neither active at the time of investigation nor before in gymnastics, either as athletes, judges or coaches (age: 30.50 ± 14.42). The study was conducted in compliance with the Declaration of Helsinki for Human Research and the International Principles governing research on humans, as well as in compliance with the ethical guidelines of the local ethics committee (2019.05.09_eb_10).
Instruments and Material
Video recordings
The video sequences from which the kinematic data were extracted and the stimuli for the computer-based paired comparison perception task were generated showing different handstand - back handspring performances on the balance beam (height = 1.25 m) of ten female near-expert gymnasts (age: 11.50 ± 1.43; body height: 143.00 ± 11.36 cm) with an average training volume of 26 hours per week (Figure 1). The criteria to choose this element combination were that it is often presented in competition and is therefore well known by gymnasts, coaches and judges, and that because of the restricted movement area, the spatial movement variability is low. The video recordings were taken with a digital camera (240 Hz, 1920 x 1080 pixel) at a distance of 15 m from the beam from the judge´s perspective (viewed on a sagittal plane). The video sequences were cut to the desired duration of the movement from the beginning of the initial movement to a stable landing position. For the experiment, eleven handstand - back handspring trials out of a sample of 60 were chosen with the criterion of a wide range of kinematic patterns and movement quality. Therefore, the kinematic pattern (the same procedure as described in the section Movement pattern analysis) as well as the movement quality were ascertained for all 60 trials in advance. To determine the movement quality, the 60 videos were rated by three gymnastics experts (visual and motor experience in research and praxis for over ten years) on a visual analog scale that was anchored to six points according to the judgment guidelines of the German Gymnastics Federation for young gymnastics talents (DTB, 2001): (1) technically poor execution or fall, (2) technical errors with large postural errors, (3) technical errors with minor postural errors, (4) good execution with minor postural errors, (5) good execution, and (6) very good execution. To evaluate the reliability of the scores, an intraclass correlation was calculated (ICC = 0.729). The average rating of the videos was 3.40 ± 1.48, the cluster analysis resulted in four clusters. For the experiment, out of each cluster the trial with the best rating, and the trial with the worst rating was chosen. Additionally, out of the three clusters which contained the highest amount of trials, the trial which was nearest to the mean rating of the trials of the cluster was taken.
Movement pattern
To determine the kinematic movement pattern, the horizontal and vertical coordinates of 14 points (body landmarks, representing the ankle, knee, hip, shoulder, elbow, and wrist joints of the left and right body sides as well as the head and the tailbone) were digitized for each frame of the video sequences with the movement analysis software Simi Motion®. A software built-in digital filter was applied for data smoothing. Each of the body landmarks was represented by two-dimensional time series [xj(t); yj(t)] with j = 1, 2, 3,..., j (t = time, j = frame number). The time series of the body landmarks were time normalized and rescaled to the interval [0; 1000] for each trial. Time courses of the body angles for the knee, hip and shoulder joints (left and right body side) as well as the trunk orientation angle were calculated.
The kinematic pattern was calculated using a cluster analysis (Mack et al., 2018). For each time course of joint angles as well as the trunk orientation angle between each pair of two handstand - back handspring trials, Euclidean distances were calculated and summed to one Euclidean distance value for each pair of two handstand - back handspring trials. A value of zero indicated an identical course, whereas the larger the resulting value, the more dissimilar two trials were. The resulting Euclidean distance matrix was evaluated by a hierarchical cluster analysis using Ward's hierarchical clustering method (Ward, 1963).
Perceived movement quality
The perceived movement quality was analyzed using a paired comparison test. Whenever a video was judged to be of better quality than its counterpart, it was scored with one point. If both videos were judged of equal quality, neither was scored with a point. This procedure was performed for all results of the 66 paired comparisons (of the eleven video sequences) of each of the 30 participants who performed the paired comparison test. The resulted values were summed up over the participants of each experimental group, resulting in three Euclidian distance matrices. This approach is similar to the SDA method (Lander, 1991; Lander and Lange, 1996), the SDA-M method (Schack, 2004) or the Repertory Grid Technique (Fransella et al., 2004), which are used to identify the way people construe a particular experience. The results were structured with a cluster analysis and visualized as a tree diagram to compare the resulting tree diagram of the kinematic pattern and the perceived movement quality of the three different experimental groups. Design and Procedures
Figure 2 shows a schematic representation of the whole study protocol. All participants were asked for their written agreement and level of experience before their participation, which was voluntary. In the first step the 60 video sequences were recorded. The kinematics and the movement quality of the handstand - back handspring performances of ten gymnasts were structured and analyzed. Eleven videos were chosen for the second step of the study. Next, other 30 participants performed the paired comparison test. Thereby, each of the eleven video sequence was compared with each other video sequence, but not with itself, yielding 66 comparisons. Participants were instructed to decide whether the movement quality was better for the first presented video sequence, the second presented video sequence or whether both video sequences showed the same movement quality for each comparison. The order of the trials was randomized for each participant within the experiment.
Statistical Analysis
The Euclidean distance matrices of the kinematic pattern and the perceived movement quality of the three experimental groups were normalized and compared in R using the dendextend R package (Galili, 2015). Therefore, the Baker’s Gamma Index, which is a measure of the association between two dendrograms of a cluster analysis, was calculated (Baker, 1994) for all six combinations. Therefore, the merging, but also the height of the branch in which they merged, was taken into account. The value ranges between -1 and 1. A value near zero implies that the two tree diagrams are entirely dissimilar.
Results
Figure 3 shows the tree diagram of the kinematic pattern of the eleven performances of the handstand - back handspring as well as the resulting pattern of the paired comparison test concerning the perceived movement quality of the three experimental groups.
Figure 3
The tree diagrams resulting from a cluster analysis using Wards’ clustering algorithm for a) the kinematic pattern of the respective gymnastics skills as well as the resulting pattern of the paired comparison test concerning the perceived movement quality of b) the motor experts, c) the novices and d) the visual experts. Note: The y-axes of the dendrograms represent the measure of closeness (similarity) of individual trials and clusters. The dashed line represents the Euclidean distance below which the clusters are identified.
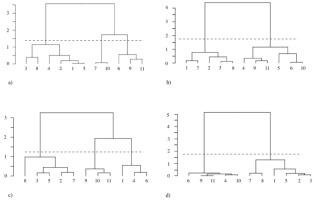
Analysis of data concerning the number of subgroups (i.e., clusters) with the elbow method and the silhouette method revealed two clusters for the dendrogram of the visual and motor experts and three clusters for the dendrogram of novices and the kinematic pattern. Differences between the diagrams of the three experimental groups and the kinematic pattern could also be found regarding the height of the branches in which they merged as well as the distribution of the trials to two different clusters. The height of the branches represents the similarity of the results of the paired comparison test for participants of a particular experimental group.
The Baker’s Gamma Index revealed no evidence that the dendrogram of the kinematic pattern was similar to the dendrogram of the perceived movement quality of visual experts (r = .35, p = .143), motor experts (r = .14, p = .339) and novices (r = .15, p = .329). Concerning the comparison of dendrograms of the perceived movement quality of the different groups, there was a significant correlation between the dendrogram of visual experts and motor experts (r = .62, p = .021), as well as a significant correlation between the dendrograms of visual experts and novices (r = .68, p = .011). There was no significant correlation between the dendrograms of motor experts and novices (r = .32, p = .173).
Discussion
The study aimed to investigate the relationship between the perceived movement quality of a gymnastics skill and its kinematic pattern, as well as the influence of expertise on this relationship. The study built on previous research that has found links between the mental representation of a movement and the movement kinematics (Schack, 2003) together with the results of studies concerning the perceptual significance of certain structural and kinematic aspects in movements when identifying and evaluating human motion (Troje, 2002). While there is an expanse of research investigating the order and structure of functional and sensory features of mental representations, the question remains whether all those features were taken into account equally when evaluating the quality of a particular movement as well as the role of expertise in those perceptual and cognitive processes.
A comparison of the relationship between the cluster solutions of the three experimental groups with the kinematic pattern revealed no significant correlation. Therefore, it could be presumed that different kinematic aspects have a different weight in the judgment process. A bent knee angle could, for example, decrease the judgment score much more than a bent hip angle or the landing position could influence the judgment score more than the starting position. The influence of different kinematic variables on the movement quality varies also for the different elements. A bent knee in a handstand is weighted in a different way than a bent knee in a back handspring. It could also be presumed that the quality evaluation is based not only on kinematic features, but also other variables. Several studies showed that judges not only base their rating on the basis of kinematic features, but also consider subtle features such as dress, facial expression, mental capacities, personal experiences (Plessner and Haar, 2006), expertise (Heinen et al., 2013; Pizzera, 2012; Pizzera and Raab, 2012; Ste-Marie, 1999), as well as the point of view (Plessner and Schallis, 2005) and the order of presentation of the gymnasts (Ansorge et al., 1978; de Bruine, 2006). There is still a lack of research investigating the different weighting of particular aspects in the process of quality evaluation and the here used method cluster analysis might have some limitations to investigate this particular topic. To gain insight into those variables that affect quality judgment, analyzing methodologies such as neural network algorithms could be taken into account.
A comparison of the relationship between the tree diagrams of three experimental groups revealed a significant correlation between the dendrograms of visual experts and motor experts, as well as between the dendrograms of visual experts and novices, but no significant correlation between the dendrograms of motor experts and novices. It could be assumed that those differences emerged because the different gymnastics expertise level leads to differences concerning the importance of particular kinematic aspects. Perhaps, for motor experts, kinematics aspects, which are relevant to perform a movement in a certain way, are of central importance, and for novices, the clearly visible quality-determining kinematic aspects. Visual experts, such as coaches and judges, have to keep the movement guiding kinematic aspects in mind as well as the quality-determining kinematic aspects. These results are in line with research on visual search strategies while evaluating gymnastic movements. It could be shown that different levels of gymnastics expertise lead to a different perceptual pattern (focusing on various information sources) as well as different judgments (Bard et al., 1980; Del Campo and Gracia, 2018; Pizzera et al., 2018).
When looking at the number of clusters of the dendrograms of the three experimental groups, two clusters could be identified for motor experts as well as visual experts, and three clusters could be recognized for novices. However, not only differences concerning the number of clusters, but also the closeness of different clusters was found. The two distinct clusters of the dendrograms of visual and motor experts were less close than the three different clusters of the dendrograms of novices. This finding suggests that there was more variance in the decision regarding the movement quality for novices than for visual and motor experts. This difference could occur for two reasons. First, it could be that the representation of the kinematic movement pattern of novices was not as detailed as for visual and motor experts, or second, they had a detailed representation of the kinematic pattern, but the representation of what should be rated as good or bad quality was not that detailed.
When looking at the results, it could not be stated whether the perception of the movement or the different weighting of the kinematic aspects led to the differences in the dendrograms of motor experts and novices. Therefore, it would be interesting to compare the mental representation of a certain gymnastics skill in terms of their functional states and sensory events (e.g., Schack, 2003) with the pattern of the perceived movement quality. It could be assumed that the mental representation has a mediating influence on the evaluation of the quality of a movement and the quality-influencing variables.
The study revealed differences between gymnastics visual experts, motor experts and novices regarding the pattern of the perceived movement quality of the gymnastics skill handstand - back handspring. The pattern of perceived movement quality was not correlated with the holistic kinematic pattern of the judged skills. The results suggest perceptual and cognitive differences for the participants when evaluating gymnastics skills based on their different previous visual and motor experience. Investigating the relationship between the pattern of the perceived movement quality and the kinematic pattern may be useful for diagnosing the objectivity of the evaluation of movement quality or guiding judging training. Coaches could set a priority on these aspects in training, and judges could keep their own prioritization in mind to avoid a subjective judgment.