Introduction
The versatility of strength training contains a comprehensive selection of exercises (single and multi-joint). Recent studies have identified different ways to increase muscle recruitment during strength training, including the use of verbal instructions (Fujita et al., 2019, 2020), exercise order (Gentil et al., 2007), and volume of repetitions (Paz et al., 2020). A possible strategy to increase muscle recruitment could be muscle pre fatigue, and in this sense muscle pre-exhaustion (PreEx) was proposed (Jones, 1970). In contrast to frequent strength training which is usually composed of multi-joint exercises which involve larger muscle groups in order to enhance the effects of training (Fleck and Kraemer, 2014), the PreEx method aims to increase muscle recruitment of the target muscle by pre-fatiguing it through a uniarticular exercise immediately before a subsequent multiarticular exercise (Brennecke et al., 2009).
Previous studies have shown controversial results regarding the effects of PreEx on electromyographic (EMG) activity of the target muscle. Augustsson et al. (2003) showed a decrease in the EMG activity of the vastus lateralis and rectus femoris muscles in the leg press exercise performed after PreEx by the leg extension exercise. In contrast, other studies have shown that PreEx increased the EMG activity in target muscle (Pirauá et al., 2017; Rocha Júnior et al., 2010). Some studies have also shown an increase in EMG activity in accessory muscles, without alteration of agonist muscles (Brennecke et al., 2009; Gentil et al., 2007; Golas et al., 2017). Recently, Ribeiro et al. (2019) concluded in a review of the literature that PreEx does not increase EMG amplitude in the target muscle, but can enhance EMG amplitude of synergistic muscle.
It is worth mentioning that all these previous studies examined the PreEx effect on EMG activity by looking at all repetitions together. However, due to the muscular fatigue that is developed as the repetitions occur, it can be expected that PreEx may have a different influence throughout the subsequent repetitions. In this way, a more detailed analysis at different intervals throughout the set of repetitions could provide new insights regarding the effects of PreEx on muscle recruitment. To the best of our knowledge, the study of Soares et al. (2016) was the only study that investigated the PreEx effect at different intervals. Those authors analyzed the EMG activity of the first and the last repetition of the triceps brachii muscles in the bench press exercise performed before and immediately after PreEx by the triceps push down exercise. The results showed no difference in the triceps brachi EMG activity between conditions with or without PreEx in both repetitions (Soares et al., 2016). However, we believe that further studies involving subjects with different levels of training experience, other muscle groups, and considering different intervals throughout the set of repetitions are still needed to substantiate the understanding regarding the PreEx influence on muscle recruitment.
Even with substantial amounts of paper regarding the effects of PreEx on EMG activity, all these previous studies have analyzed the EMG data only in the temporal domain. The effects of PreEx on the EMG frequency domain are still unknown. Thus, performing spectral analysis could reveal the behavior of fatigue during exercises performed in this training method (Contessa et al., 2016). Furthermore, even though it is well established that EMG frequency declines through maximal isometric efforts (Gandevia, 2001), and also in dynamic isokinetic repetitions (Komi and Tesch, 1979), it is still unknown whether PreEx has any influence on the firing frequency of motor units. Analysis in the spectral domain could add relevant information regarding the effect of PreEx on muscle recruitment. This information would help understand whether this method really allows to train the target muscle harder than is normally possible in traditional sets.
Therefore, this study aimed to verify the effects of the PreEx method on EMG activity (especially in the frequency domain) among different intervals of repetitions in the seated row exercise. Based on previous studies, we hypothesized that in the PreEx method the median frequency of the analyzed muscles would decrease throughout the sets (intermediate and final repetitions). Additionally, we expected that the EMG root mean square (RMS) of the target muscle (latissimus dorsi) would not increase.
Methods
Participants
A total of 20 male adults (19.80±1.54 years; 71.92±9.39kg; 177.28±07cm) participated in the study. Eleven participants reported little (4.78±1.30 months), and nine reported no previous experience in strength training. No participant was involved in any exercise program within one year before the study. Exclusion criteria were: a) to be engaged in strength training within the past 12 months, b) to present any orthopedic problem in the last six months, c) to present any neurologic problem, or d) any other health issue that would make it impossible to perform the proposed exercises. The local Ethics Committee, which followed the Declaration of Helsinki, approved all the experimental procedures.
Design and Procedures
Each subject visited the laboratory twice with a minimum rest of two days. None of the sessions exceeded two hours.
Load quantification test
In the first session, the one-repetition maximum (1RM) of the participants was estimated using the Brzycki (1993) equation. Initially, participants were instructed regarding the movement for the seated row exercise and the pullover exercise. Subsequently, participants warmed-up with two sets of 12 submaximal repetitions in the seated row exercise and pullover exercise, respectively. Between each set, there were 90 s of passive rest. After the warm-up, participants rested for five minutes, and subsequently performed a load quantification test. For both the seated row exercise and the pullover exercise, participants performed the maximum number of repetitions until failure. Between each load quantification test participants rested for five minutes. The protocol proposed by Lesuer et al. (1997) was followed, which recommends a range of 6 to 10 repetitions to ensure the reliability of load estimation. In the few situations when participants performed more repetitions than recommended, a five minute rest interval was given before the next attempt with a greater load. The cadence of exercise movement was set at two seconds for the eccentric and two seconds for the concentric phase, controlled by a metronome.
EMG sensor placement and data collection
In the second session, the EMG data were collected using surface wireless electrodes (Trigno Wireless, Delsys, Boston, USA) at the sampling frequency of 2000Hz. The electrodes were positioned over the latissimus dorsi (LD), teres major (TM), biceps brachii (BB), and posterior deltoid (PD) muscles. In the LD, the electrode was positioned obliquely 4cm below the lower angle of the scapula (Escamilla et al., 2006). In the TM the sensors were fixed in the middle of the muscle bundle along the scapula’s lateral edge (Konrad, 2005). For the BB the sensor placement followed SENIAM procedures (Hermens et al., 2000). In the PD, the sensors were placed approximately 4cm behind the angle of the acromion (Schoenfeld et al., 2013). A single researcher placed all electrodes on the dominant side of the participant’s skin.
Maximal voluntary isometric contraction
Subsequent to the placement of the sensors, participants warmed-up with two sets of 12 repetitions of the seated row exercise at 40%1RM following the same cadence and rest interval used in the load quantification test. In sequence, participants performed maximum isometric voluntary contractions (MIVCs) (Figure 1).
Figure 1
Participants’ positioning during maximum isometric voluntary contraction for the (A) latissimus dorsi and teres major, (B) posterior deltoid, (C) biceps brachii.
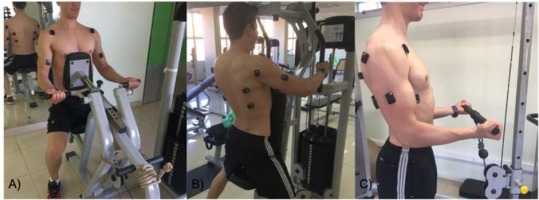
The MVICs for the LD and TM were measured on the seated row machine. Participants remained with their upper limbs by the trunk, maintaining their forearm in the supine position, and their elbows flexed at 90º (Lehman et al., 2004). The MVIC of the PD muscle was measured with horizontal abduction of shoulders on the peck deck machine. The shoulders were maintained at 90º of horizontal adduction with the wrist in a neutral position (Schoenfeld et al., 2013). Finally, the MVIC of the BB muscle was measured on a dual adjustable pulley machine with the elbow flexed at 90º, maintaining the wrist in the supine position (Alenabi et al., 2013). The angles of all joints were measured with a goniometer. In all MVIC measures, participants were verbally motivated to perform the movement at their maximum effort. For each muscle, participants performed three efforts of 5s MVIC, with 90s rest intervals in between.
Pre exhaustion effect
After EMG acquisition during the MIVC contraction, participants rested for five minutes and then the PreEx effect on EMG activity was measured. Participants performed in a counterbalanced form (interspersed order), two sets of the seated row exercise (one with and another without PreEx). The condition to be performed first was decided by a raffle, using an electronic app (Coin Flip Plus – iHandy Ltd., Kowloon, Hong Kong, China). In the PreEx condition, the seated row exercise was performed immediately after the pullover exercise (Figure 2). All exercises were performed with an intensity of 70%1RM. The interval between the conditions was 20 minutes (Augustsson et al., 2003). During both sets participants were encouraged to achieve concentric muscle failure.
EMG analysis
The EMG data were digitally filtered with a fourth-order zero-lag Butterworth filter (bandpass 10-500Hz). For analysis of MVIC the first and the last second were excluded (Fujita et al., 2020), as well as the first and the last repetition of the seated row sets, since these periods were more susceptible to mistakes in cadence and movement execution in the exercises (Gentil et al., 2007).
Considering the three different temporal windows (two initial repetitions, two intermediate repetitions, and two final repetitions) of both sets of the seated row exercise, we calculated the magnitude of EMG activity by measuring the RMS. Each interval RMS value was subsequently normalized by the average of RMS of the three MVIC for each muscle.
Additionally, the median frequency was chosen for spectral analysis because it is less sensitive to noise (De Luca, 1997). The same temporal windows were analyzed for the frequency domain of EMG activity. We conducted a spectral analysis to obtain the power spectrum density of the EMG activity using Welch’s method. These variables were computed using customized scripts created in MatLab R2019a (Mathworks Inc., Natick, MA, United States).
Statistical Analysis
As all variables presented normal data distribution and homogeneity of variance, eight two-way ANOVAs with repeated measures were performed (PreEx vs. Interval of repetitions). The dependent variables were RMS and median frequency of each muscle. When necessary, the Bonferroni post hoc tests were applied. The level of significance was set at p<0.05 for all analyses.
Results
RMS analysis
For the LD muscle, ANOVA indicated an effect for the interval of repetitions [F2,38=5.508, p=0.008] and an interaction between PreEx and the interval of repetitions [F2,38=6.183, p=0.005]. Post hoc tests indicated that EMG activity of the LD increased in the intermediate and final repetitions without PreEx.
Regarding the TM muscle, ANOVA showed an effect for PreEx [F1,15=11.435, p=0.004] and the interval of repetition [F2,30=6.846, p=0.004]. The TM RMS was higher without PreEx. Furthermore, the EMG activity was higher throughout intermediate repetitions.
For the BB muscle, ANOVA revealed an effect for the interval of repetitions [F2,38=26.177, p=0.000]. Post hoc tests indicated that the BB RMS increased in the intermediate and final repetitions.
Finally, for the PD muscle, ANOVA showed an interaction between PreEx and the interval of repetitions [F2,38=5.262, p=0.010]. Post hoc tests indicated that PD RMS increased in the intermediate repetitions without PreEx.
Spectral analysis
Regarding the LD muscle, ANOVA showed an effect for the interval of repetitions [F2,38=31.356, p=0.000]. Post hoc tests indicated that LD median frequency reduced in the intermediate and final repetitions, and there was a reduction in the final repetitions compared to intermediate repetitions.
For the TM muscle, ANOVA showed an effect for PreEx [F1,19=10.847, p=0.004], and the interval of repetitions [F2,38=31.020, p=0.000]. Additionally, there was an interaction between PreEx and the interval of repetitions [F2,38=4.302, p=0.021]. Post hoc tests indicated a decrease in the TM median frequency in the intermediate and final repetitions for both exercise conditions. There was also a decrease in the final repetitions compared to intermediate repetitions without the PreEx condition.
Regarding the BB muscle, ANOVA indicated an effect for the interval of repetitions [F2,38=18.597, p=0.000]. Post hoc tests showed a decrease in the BB median frequency in the intermediate and final repetitions. There was also a decrease in the BB median frequency in the final repetitions compared to intermediate repetitions.
For the PD muscle, ANOVA showed an effect for PreEx [F1,19=13.862, p=0.001] and the interval of repetitions [F2,38=73.536, p=0.000]. The PD median frequency was reduced in the PreEx condition. Post hoc tests also showed a decrease in PD median frequency in the intermediate and final repetitions. Finally, there was a reduction in PD median frequency in the final repetitions compared to the intermediate repetitions.
Figure 3
Root mean square as a percentage of maximum isometric activity of the A) latissimus dorsi, B) teres major, C) biceps brachii, and D) posterior deltoid muscle during initial, intermediate, and final repetitions of the seated row exercise with and without pre-exhaustion.
▲ denotes difference between pre-exhaustion conditions
#denotes a significant difference from initial repetitions p<0.05
*denotes a significant difference from other intervals of repetitions p<0.05
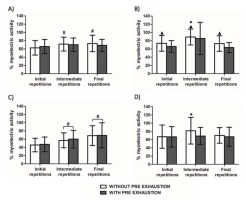
Figure 4
Median frequency of electromyographic activity of the A) latissimus dorsi, B) teres major, C) biceps brachii, and D) posterior deltoid muscle during initial, intermediate and final repetitions of the seated row exercise with and without pre-exhaustion.
▲denotes a significant difference between pre-exhaustion conditions
#denotes a significant difference from initial repetitions p<0.05
✤ denotes a significant difference from intermediate repetitions p<0.05
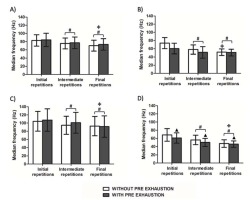
Discussion
The present study aimed to verify the effects of the PreEx method on EMG activity (RMS and median frequency) among different intervals of repetitions in the seated row exercise under two different conditions (with vs. without PreEx). The main findings revealed that PreEx did not increase the RMS of the target muscle in any interval of sets. Additionally, the PreEx condition decreased the PD median frequency. Finally the results showed that the firing rates (median frequency) were reduced for all analyzed muscles during intermediate and final repetitions under both exercise conditions.
PreEx is based on the hypothesis that in multi-joint exercises, the point of momentary muscular failure occurs when the weakest muscles involved in the exercise are no longer able to apply the required force to maintain the exercise (Jones, 1970). Thus, PreEx was proposed in an attempt to pre-exhaust the target muscle using single-joint exercises before a multi-joint one, as it was believed that PreEx could fatigue the agonist muscle, leading to higher muscle activation during subsequent multi-joint exercise (Fisher et al., 2014). Even though some studies have shown positive effects of PreEx to increase EMG activity in target muscles (Pirauá et al., 2017; Rocha Júnior et al., 2010), important factors such as exercise intensity and exercises completed before concentric failure need to be highlighted in these studies. Rocha Junior et al. (2010) applied PreEx with low intensities (30 and 60%1RM) in a single-joint exercise of leg extension before the leg press exercise. Those authors showed higher values in vastus lateralis RMS (67.36% for PreEx at 30%1RM; and 59.46% for PreEx at 60%1RM) compared to conventional sets (27.61%). Likewise, Pirauá et al. (2017) showed that PreEx increased the pectoralis major and anterior deltoid EMG activity by 32.21% and 7.81%, respectively. Their participants performed the single-joint exercise (dumbbell fly) with a low intensity of 30%1RM before the barbell bench press exercise. In both protocol studies, participants performed the multi-joint exercise with a low intensity of 60%1RM, and generally did not execute the sets until failure as proposed by Jones (1970). These factors could contribute to generate the positive effects of PreEx.
In contrast, our results revealed that the EMG activity of the target muscle (LD) did not increase when performing a single joint exercise before a multi-joint exercise. Our findings corroborate previous studies (Brennecke et al., 2009; Gentil et al., 2007; Golas et al., 2017) and the lack of differences was likely found due to the intensity of the exercise. The moderate intensity of 70%1RM used in the seated row exercise required a greater EMG activity (69.24%) of the LD target muscle during the seated row exercise without the PreEx condition, while in previous studies, the EMG activity was 27.61% (Rocha Júnior et al., 2010) and 37.53% (Pirauá et al., 2017) in the target muscle during the exercise sets without PreEx. These differences probably exist due to the different intensities used in the protocol studies. When the exercises are performed with greater loads, more motor units are recruited to perform the movement (Calatayud et al., 2016), thus under both conditions the greater load used in our study required greater EMG RMS, but with no differences being shown between them. Another possible explanation for these divergent results could be related to differences in the participants’ strength training experience. While previous studies considered subjects with strength training experience of at least six months (Pirauá et al., 2017; Rocha Júnior et al., 2010), we enrolled untrained individuals. It could be argued that untrained individuals present less intra and intermuscular coordination (Bompa and Buzzichelli, 2015), and for this reason, the exercise performed at an intensity of 70%1RM already demands maximum muscle activation even without pre-exhaustion. Our results are pioneers in indicating that the PreEx method does not increase target muscle recruitment in untrained subjects. Furthermore our results corroborate Soares et al. (2016) showing that the EMG activity of the target (LD) and accessory muscles (BB) was greater in the final repetitions compared to initial ones. Moreover, our results add information regarding the intermediate repetitions, showing that EMG activity increased in the midle of the strength training set.
Regarding the median frequency, this was the first study to analyze the spectral domain of the EMG signal during PreEx sets. As the literature shows, changes in the frequency domain of EMG accompany muscle fatigue (Gandevia, 2001), and generally the presence of muscle fatigue induces a reduction in the firing rate (Contessa et al., 2016). It could be expected that during initial repetitions during the PreEx condition, the fatigue level could be greater than without PreEx. In this sense, since the prime mover of the pullover exercise is the shoulder extension, it would be expected that the LD and the TM muscles would present decreases in the median frequency in the beginning of the seated row exercise (performed immediately after the pullover exercise). However, our results indicated that PreEx only influenced the firing rates in the PD muscle, which reduced compared to the non-PreEx condition. A possible explanation is muscle synergy, in which the central nervous system controls specific muscle groups to perform the movement (Ghislieri et al., 2020). In this sense, probably the posterior deltoid and other agonist muscles for shoulder extension, such as the triceps brachii long head, could have higher participation in the pullover exercise. Although we did not analyze EMG activity from the triceps brachii long head, a previous study (Borges et al., 2018) revealed that the triceps brachii long head showed higher EMG activity (34.3%) than the LD muscle (22.7%) during the pullover exercise. Considering muscle synergy theory, maybe our participants emphasized the PD and triceps brachii long head to perform the pullover exercise instead of recruiting the LD and TM preferably. Furthermore, our results regarding the median frequency showed that in the intermediate and final repetitions, the median frequency was lower than initial repetitions for all muscles, and under both conditions (with or without PreEx). Thus, based on our findings, we can assume that both conditions generated muscle fatigue in the LD, TM, BB, and PD muscles in the final repetitions compared to initial repetitions.
The results of the present study also showed that the number of repetitions performed by participants in the seated row exercise was lower (8.75±2.02) during the PreEx condition compared to non-PreEx (11.70±2.43). This finding corroborates other studies showing that PreEx decreases the number of repetitions by ~20% during the second exercise (Soares et al., 2016) compared to a conventional set. Augustsson et al. (2003) showed that participants executed fewer repetitions (7.9±1.4) of the leg press exercise with PreEx (leg extension) compared to the leg press without PreEx (9.3±2.3). Additionally, Gentil et al. (2007) showed that when the chest press exercise was preceded by the peck deck exercise, the number of repetitions was reduced from 9.50±0.80 to 5.33±1.15. There seems to be a consensus that PreEx decreases the number of repetitions in the multi-joint exercise during PreEx sets.
Some studies have mentioned that if the main exercise is multi-joint, it should be performed first in the training set or session (Nunes et al., 2020; Senna et al., 2019). In a recent review of the literature, it was found that the exercise order influences gains in muscular strength, mainly in those exercises performed at the beginning of a training session (Nunes et al., 2020). Considering these studies, the decrease in the number of repetitions during the PreEx condition, and the lack of the EMG RMS difference between conditions in the present study, we could state that PreEx does not seem to be an interesting strategy to promote greater hypertrophy and strength compared to the traditional method of resistance training. Furthermore, a recent study showed that PreEx had similar effects to conventional strength training on muscle strength and hypertrophy after nine weeks of intervention (Trindade et al., 2019). The authors showed that both methods caused similar gains for strength and hypertrophy, but only participants of the PreEx group showed hypertrophy in the proximal region of the vastus lateralis muscle when compared to the control group.
Conclusions
According to our results, we may conclude that the PreEx method seems ineffective in increasing the EMG RMS of the target muscle throughout all repetitions of one strength training set. Also, the PreEx exercise used in our study seems to be a good means to increase fatigue in the posterior deltoid muscle. However, there are potential limitations of this study which can influence the statement that the PreEx method does not seem to be a preferred way to increase the EMG activity of the target muscle for untrained individuals. First of all, we could have analyzed the non-dominant limb EMG activity once a previous study showed a significant difference between the two sides (Golas et al., 2018). Also, it is important to note that the present study evaluated the EMG activity only during one acute session. For a better understanding of the PreEx effect on EMG activity, especially for the median frequency, future studies could verify the EMG activity in longitudinal studies. Furthermore, future research can observe muscle synergies during PreEx. Lastly, we could have included analyzing the movement's external kinematic and kinetic structure to verify whether changes in velocity, acceleration, force, and torque values, for example, during the exercise would occur (Król and Gołaś, 2017).