Introduction
Soccer is an intermittent sport, characterized by periods of high-intensity bouts including changes of direction, jumps and sprints interspersed with lower intensity actions or passive recovery such as walking. The energy production is mainly dependent upon both aerobic (Mcmillan et al., 2005; Schippinge et al., 2002) and anaerobic (Bangsbo et al., 2006) metabolism. This sport with many explosive actions is played at approximately 80-90% of the maximum heart rate or 70-75% of maximum oxygen uptake (VO2max) (Bangsbo, 1994). Physiological responses to soccer training, with a large number of explosive actions and strong eccentric components (Nédélec et al., 2012), include post-exercise muscle damage (Hoff and Helgerud, 2004; Nédélec et al., 2004) and an increase in reactive oxygen species (ROS) in the blood and at the mitochondria of skeletal muscle (Viguie et al., 1993).
These mechanisms promote exercise-induced adaptations with a lower oxidative stress level in elite athletes compared with sedentary subjects, due to the improvement of the antioxidant response as a consequence of soccer training (Brites et al., 1999). Nevertheless, an exhaustive training program with insufficient recovery could lead to an excessive ROS production compromising the antioxidant status, leading then to oxidative stress (Finaud et al., 2006a), which causes a series of different responses, such as lipid peroxidation of biological membranes leading to extensive cellular damage (Chance et al., 1979), changes in membrane fluidity, and modifications of certain antioxidants altering the redox status of the cell (Harris, 1992). Moreover, oxidative stress is markedly up-regulated by a soccer game, probably as a part of the exercise-induced inflammatory response, and is accompanied by a marked deterioration of anaerobic performance for as long as 72 hours; thus, soccer games and soccer-specific running protocols impact the oxidative stress responses in male players (Ascensão et al., 2008; Ispirlidis et al., 2008; Kingsley et al., 2005; Svensson and Drust, 2005). This highlights the importance of conserving an optimal antioxidant and redox state through the entire season in order to maintain optimal soccer performance.
The whole season in competitive soccer includes micro-cycles consisting of training, taper, competition, and recovery (Cross et al., 2019). Professional clubs may train 5-6 days during a weekly micro-cycle. Furthermore, the demand for playing 1 to 2 games per week elevates stress imposed on players, thereby increasing the injury risk and a performance decline due to fatigue, muscle damage, inflammation and oxidative stress (Perrone et al., 2019; Silva et al., 2018). Increased oxidative stress caused by exhaustive training and games may compromise exercise performance of players during a long season and especially during times with the most intensive match schedule (Ascensão et al., 2008). However, little is known about the response of antioxidant and pro-oxidant blood markers following several months of competition and soccer training characterized by multiple-sprint and intermittent-type exercise (Finaud et al., 2006a; Le Moal et al., 2016).
Research is sparse with respect to the effect of different loads on the antioxidant status changes of professional soccer players after several weeks of competition and its relation to performance (Le Moal et al., 2016); suggesting the need of monitoring main antioxidant blood markers such as the total antioxidant status (TAS), including non-enzymatic systems, and the ratio of reduced glutathione (GSH) and glutathione disulphide (GSSG), which may provide interesting information on the physiological effects of soccer games and its relation to performance. Therefore, the main aim of this study was to assess the changes of circulating levels of antioxidant blood markers before and after a mid-season of professional soccer players from three different teams belonging to the 3rd Spanish Division, and to correlate antioxidant markers with the competitive performance of each soccer team.
Methods
Design
Sixty-five male soccer players from three different soccer clubs from the province of Cadiz (Spain) were included in the study. The teams maintained their normal soccer training program. The assessment of body composition, maximal aerobic capacity and antioxidant status was performed in the first week of the championship season (pre-test) and after 18 weeks in the mid-season (post-test). Through a personal interview, participants were instructed to avoid any complementary physical exercise during the soccer training season, maintaining habitual dietary, hydration, and sleep habits. Soccer performance was registered according to the official classification ranking (3rd Spanish Division) at both the mid-season and at the end of the competitive season. The ranking position just in the mid-season and at the end of the season were 2nd and 1st for Team A, 5th and 5th for team B and, 12th and 14th for team C, respectively.
Participants
This study involved a group of 65 male soccer players (mean age of 25.3 ± 4.2 years) from three different soccer clubs of the third Spanish Division: Team A (n = 23), Team B (n = 21) and Team C (n = 21) (Table 1). All of them attended an average of 6 practices per week and a weekly official game. Participants were fully informed about the protocol and written informed consent was obtained from each player before the study commenced. Participants were free to withdraw from the study without any consequences at any time. Along the study period, all participants were healthy based on an orthopaedic and physical examination by the team physician, and their medical history assessed through a questionnaire. All procedures were approved by an Institutional Ethics Review Committee of the Cádiz University, following the current national and international laws and regulations governing the use of human subjects (Declaration of Helsinki II).
Table 1
Participants’ characteristics by team groups before the onset of the competitive season.
Body composition
Body height was measured using a wall-mounted stadiometer (Seca 222, USA) recorded to the nearest millimetre. Body mass was measured to the nearest 0.1 kg using a medical scale (Tanita, TBF-612, Japan). Fat mass, fat-free mass and the percentage of body fat were estimated using foot-to-foot bioelectric body composition analyser (Tanita, TBF-612, Japan) on an empty stomach.
VO2max Measurement
VO2max was determined through an incremental-treadmill exercise test until voluntary exhaustion on a treadmill (HP Cosmos LE300C, Sports & Medical, Nussdorf-Traunstein, Germany). VO2 was measured continuously by open-circuit spirometry and averaged every 30 s with the use of an automated online pulmonary gas exchange system via breath-by-breath analysis (Breezesuite CPX, MedGraphics, St Paul, Minnesota, USA). After 5 min of light running at a self-selected pace, the exercise protocol started at 10 km/h and increased in speed by 2 km/h every 2 min with 0% of gradient in step 1 and 2, a 2.5 % of gradient in step 3 and a 5% of gradient in the next steps; this protocol was based on a previous study in professional soccer players (Jones et al., 2013) and is presented in Figure 1. The CO2 and O2 analysers were calibrated before each test using a two-point measure: a calibration gas (CO2 5%, O2 16%, N2 balance) and a reference gas which consisted of room air after ATPS (ambient temperature and pressure, saturated) to STPD (standard temperature and pressure, dry). With a 12-lead electrocardiogram, the heart rate and brachial artery cuff pressure were monitored continuously during the test and for 30 min during recovery.
Blood sampling and assays
Fasting venous blood samples were drawn from the antecubital vein (5 ml). Blood samples were immediately centrifuged at 3,000 rpm during 10 min, storing the plasma at -80⍛C until further analyses (Blood bank centrifugal Drucker 611B, Pennsylvania, USA).
Total Antioxidant Status (TAS)
The TAS levels in the plasma were determined through spectrophotometric techniques (Automated analyser Hitachi 902, Japan) using a commercial diagnostic kit from Randox Laboratories (Cat. N⍛ NX2332 Randox Laboratories Ltd., UK). This method was based on the fact that metmyoglobin reacts with H2O2 to form ferrylmyoglobin. ABTS was incubated with the 194 ferrylmyoglobin to produce the radical cation species ABTS·+. This had a relatively stable blue-green colour which was measured at 600 nm. Antioxidants in the added sample caused suppression of this colour production to a degree that was proportional to their concentration. Results were expressed as mmol/L.
Reduced/Oxidized Glutathione Ratio
The GSH/GSSG ratio was assessed using the Akerboom and Sies method, slightly modified according to the cyclical reduction method DTNB-GSSG (McMillan et al., 2005). This method was based upon the GSH present in a sample in its oxidized form GSSG which was then incubated with 5,5’ acid- ditiobisnitrobenzoico (DTNB), later reduced acquiring a yellowish colour which could be assessed spectrophotometrically. The GSSG obtained was then reduced by the action of the glutathione reductase enzyme (GSSGrd) in the presence of NADPH. The resulting GSH was again oxidised, resulting in a continuous cycle where the reduction velocity of the DTNB (with its corresponding increase in absorbance) was proportional to the total quantity of glutathione (McMillan et al., 2005).
The resulting total glutathione was assessed through the enzymatic recycling process in which the GSH was oxidised by DTNB which was subsequently reduced using reductase glutathione. The contents of the GSSG were assessed by enzymatic techniques, exposing the samples to 2-vinilpiridine during 60 min which was capable of removing all the GSH. The GSH was calculated by the difference between the total glutathione and the GSSG. Finally, we calculated the GSH/GSSG ratio in the analyses, estimated through colorimetric techniques using diagnostic kits (Merck Chemicals Ltd, Germany).
Training procedures
Participants were enrolled in a soccer training program 6 times a week, with each session lasting 120 min. Soccer training focused on the development of technical and tactical skills first and then on the improvement of physical capacities. Technical skills, such as dribbling, passing, receiving, shooting, and heading were practiced for 40–50 minutes in at least 3 training sessions a week. In addition, soccer drills and small-sided games were performed with a reduced number of players (1 vs. 1, 3 vs. 3, 4 vs. 4) to work on offensive and defensive strategies and individual tactics. High-intensity training for the development of strength, running speed and agility (with and without a ball) was carried out twice a week, and 1 training session per week included soccer games or interval training for the development of aerobic capacity. A half-field game lasting 20–30 min was played at the end of the training session twice a week. Stretching exercises for the main muscle groups were executed in the warm-up and cool-down periods. The internal training load was quantified by analysing the rating of perceived exertion (RPE) of each session. Each participant’s session RPE was collected 15 min after each training session using the Borg scale-10 (Brites et al., 1999) which players were previously familiarized with. Then, RPE value was multiplied by the total duration of training (min) to represent in a single number the magnitude of the internal training load in arbitrary units (AU) (Kingsley et al., 2005). All training sessions were fully supervised, and training diaries were maintained for each group. All participants were instructed to maintain their normal daily activities throughout the study, including participation in recreational sporting activities. However, no additional training was permitted.
Statistical Analyses
Descriptive statistics (mean ± standard deviation, SD) for the different variables were calculated. The distribution of each variable was examined with the Kolmogorov-Smirnov normality test. The Mauchly test of sphericity was computed for repeated measures and the Levene’s test for independent analysis. The characteristics of body composition, VO2max at baseline and RPE values according to the soccer team were analysed through one-way ANOVA. A 3×2 mixed ANOVA was performed to assess the interactions between the Performance Level (Team A vs. Team B vs. Team C) and time (pre vs. mid-season) on antioxidant status. Post hoc comparisons were performed by the Bonferroni test. The relationship between blood markers and other characteristics with team performance (measured with the team ranking and points at the 18th week and the end of the season) was also analysed by multivariate linear stepwise regression analysis adjusted by confounding variables including age, body mass and fat percentage. The level of significance was set at p < 0.05. Statistical analysis was carried out using SPSS v.22 (SPSS Inc., Chicago, IL, USA).
Results
The one-way ANOVA by performance (Team A, Team B and Team C) showed no significant differences in any variable; therefore, these variables were not able to determine soccer performance when the complete team was analysed. Moreover, the levels of the RPE were similar among groups (Team A = 322.1 ± 11.6 AU; Team B = 316.7 ± 11.3 AU, Team C = 324.8 ± 13.2 AU) with no statistically significant differences in both total and weekly values.
However, there were differences in the analysed antioxidant markers according to the performance level, since a statistically significant interaction of performance*time for both antioxidant markers TAS (F (2, 62) = 12.19; p < 0.001; η2p = 0.315) and the GSH/GSSG ratio (F (2, 62) = 12.28; p < 0.001; η2p = 0.317) was found. Moreover, antioxidant levels showed significant main effects of performance (TAS: F (2, 62) = 10.75; p < 0.001; η2p = 0.289; GSH/GSSG ratio: F (2, 62) = 15.22; p < 0.001; η2p = 0.365), where a higher antioxidant capacity was observed in the best performance soccer team (Team A vs. Team B and Team C), both before and after the mid-season. Moreover, both TAS (F (1, 62) = 530.78; p < 0.001; η2p = 0.909) and GSH/GSSG ratio (F (1, 62) = 526.70; p < 0.001; η2p = 0.909) levels significantly decreased after the 18-week training program compared with baseline values in all soccer teams. Bonferroni post-hoc comparisons are shown in Figure 2 for TAS and Figure 3 for the GSH/GSSG ratio.
Figure 2
Total antioxidant status levels before (pre) and at the mid-season (post) of each soccer team. Data include mean and standard deviation. * p < 0.05 pre vs. post the 18 weeks of the season; # p < 0.05 vs. Team A; & p < 0.05 vs. Team B.
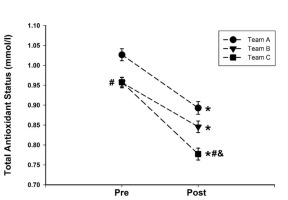
Figure 3
Reduced glutathione/Oxidized glutathione (GSH/GSSG) ratio before (pre) and at the mid-season (post) of each soccer team. Data include mean and standard deviation. * p < 0.05 pre vs. post
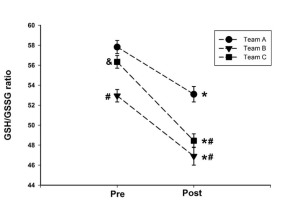
The association between performance and the analysed variables was also tested through multiple linear stepwise regression analysis by applying to models potential confounding variables including age, body mass and fat percentage. After adjustment, TAS levels after 18 weeks and VO2max were the only variables to independently predict (p < 0.05) performance (Table 2).
Table 2
Multivariate analysis: factors associated with performance (regression model)
Discussion
A novel approach in this study is that the antioxidant status was able to discriminate between performances according to the results of 3rd Spanish Division soccer teams along the season, even when measured before the onset of the season. Thus, the higher plasma levels of TAS the soccer team presented, the better performance achieved. Moreover, our results substantiated that training combined with competitions compromised the redox state of athletes (lower TAS and GSH/GSSG ratio), suggesting oxidative stress induction as a consequence of excessive exercise demands, especially in low-ranked teams.
Endurance trained athletes have lower oxidative stress and muscle damage than a sedentary population (Barranco-Ruiz et al., 2017), thus, aerobic training such as soccer training, is expected to have a positive effect on antioxidant status (Banfi et al., 2006; Margonis et al., 2007; Teixeira et al., 2009). However, along the season, physical demands could be too high since, in our study, the antioxidant levels were significantly decreased at the mid-season in all soccer teams. The decrement in the antioxidant defence system in the blood could be partly explained by the intensive training periods which players followed during the competitive season; accordingly, previous studies have reported that strenuous high intensity exercise leads to a decrease in circulating antioxidant markers (Hoff, 2005; Stølen et al., 2005). It is noteworthy to recall that the antioxidant defense system in the blood is complex in nature and represents an interaction of different components, including antioxidant enzymes and non-enzymatic antioxidants.
The GSH/GSSG ratio has been used as a marker due to its relationship with oxidative stress status in biological systems (Margonis et al., 2007) and is considered one of the most sensitive markers in response to training (Elokda and Nielsen, 2007). In the present investigation, a significant decrease in the GSH/GSSG ratio was observed after 18 weeks of training in the three teams. Accordingly, a previous study (Le Moal et al., 2016) showed a decrement in the GSH/GSSG ratio during the competitive periods in soccer players, establishing a relationship of GSH/GSSG ratio variations and accumulated training loads during in-season periods and suggesting that training intensity may alter cellular integrity. For these reasons, the significant antioxidant decrease reported in our study could be produced by the increased ROS production due to the strenuous intense training program that may alter the redox homeostasis (Kajaia et al., 2018). These results are contrary to those of other authors who found no differences in overall antioxidants through the season in rugby players (Finaud et al., 2006b). However, in our study, the decrease in the antioxidant capacity at the mid-season was also supported by plasma levels of TAS, compromising the response to exercise-induced oxidative stress.
The decrease in the antioxidant status can impact performance, since our results showed that players from successful teams (Team A) had significantly higher antioxidant levels than their less successful counterparts. Plasma TAS levels measured before the training season, were able to predict soccer performance achieved during the season, highlighting their relevance and the need of monitoring this variable in professional players. It can be explained by the fact that higher TAS levels prevent oxidative stress which has been shown to significantly increase after soccer games in male players (Ascensao et al., 2008; Bangsbo, 1994). Thus, high-ranked athletes would have a higher capacity of antioxidant up-regulation and fast mobilization of antioxidants from plasma to tissues in response to increased ROS production during exercise consequently reducing oxidative stress and damage (Vollaard et al., 2005). This fact could impact the recovery periods (Ascensao et al., 2008; Vollaard et al., 2005) and, therefore, soccer performance as it has been previously studied in other sport modalities (Braakhuis et al., 2013).
This study presents descriptive-design limitations, thus, it is possible that other variables may modulate the relationship between the antioxidant capacity and performance, such as dietary or sleep habits which were controlled through a personal interview, where athletes could have provided incorrect information affecting antioxidant markers (Barranco-Ruiz et al., 2016). Moreover, a weakness of the study is that performance was determined just by one variable (official ranking of the team), what can be influenced by several aspects such as weather, performance of the other team, etc., and, although it is a direct measure of success in competition, future research should analyse other variables that have been proposed in team sports and soccer including perceptual cognitive skills, physiological characteristics, biomechanical/technical skills, psychological characteristics and anthropometric profiles among others (Baker et al., 2020).
Our results suggest that antioxidant status is decreased after the mid-season in the professional soccer league, and it was more accentuated in the team with lower standings. Hence, this study also emphasizes the importance of keeping and maintaining high levels of aerobic capacity and antioxidant status for soccer performance during the season. Cardiovascular fitness has been related with TAS (Dokumanci et al., 2018) and was stated as one of the essential aspects of success, not only helping players to maintain their performance after several high-intensity actions during a game and the season (Stølen et al., 2005), but it has also been established as the most powerful discriminator between elite, professional and amateur players (Slimani et al., 2019). Accordingly, VO2max was a relevant factor for performance in 3rd Spanish Division soccer teams. Moreover, plasma levels of TAS were the other relevant predictor of performance and it could be related also to overtraining and potential injuries (Elokda and Nielsen, 2007; Finaud et al., 2006a, 2006b; Knez et al., 2013). Therefore, monitoring antioxidants in soccer players is needed to prevent excessive oxidative stress and cellular damage and avoid possible injuries or performance decrements.
The results presented in this study are of great interest for soccer coaches and athletes, however, it remains to be determined whether success in competition can be significantly improved due to an enhanced capacity by adjusting the training program, diet or ergogenic aids. In this line, previous studies have provided evidence that supplementation can reduce exercise-induced oxidative stress (Elejalde et al., 2021) and acidosis (Drid et al., 2016) improving recovery in soccer (Farjallah et al., 2020) in a dose-dependent manner (Al Fazazi et al., 2018) and impacting aerobic capacity (Sadowska-Krępa et al., 2017, 2019). In fact, in soccer players, a 17% higher aerobic capacity in the 28-day sesame supplementation group was reported compared with the placebo (Barbosa et al., 2017). Notwithstanding, it is important to consider that supplementation must be applied at the right and precise moment depending on the periodization of training and competitions; indeed, antioxidant supplements such as vitamin E and C, which are very commonly used by athletes, tend to block anabolic signaling pathways, and thus, impair adaptations to resistance training (Higgins et al., 2020) which are needed in soccer.