Introduction
In the United States and Japan, the number of lacrosse players has increased at all levels in recent years, from youth to post-college (Japan Lacrosse Association, 2020; US Lacrosse, 2018). Lacrosse competitions and practices are commonly held during the summer season in the United States (e.g., Major League Lacrosse) and Japan, and the upcoming World Games 2022 (Birmingham, Alabama, USA) will be held in July, in the midst of the summer season. However, when environmental conditions are hot and humid during exercise, core body temperature rapidly rises because of increased transfer of heat from the environment and inhibition of evaporative heat loss (Casa et al., 2015). Highly elevated core body temperature (>40°C) can lead to the development of heat-related illness, such as exertional heat stroke (EHS) (Casa et al., 2015). One previous study reported that the risk of heat-related illness during a boys’ lacrosse match was twice as high as that during a soccer match (Kerr et al., 2013). Since EHS is one of the leading causes of sudden death among athletes (Casa et al., 2012), an appropriate prevention strategy is needed for lacrosse players.
Lacrosse matches comprise four quarters of 15 minutes each (total 60-min) with two 2-min intervals and a 10-min half-time break. Lacrosse is considered to be one of the fastest field-based invasion games (Polley et al., 2015), and players are required to perform repeated sprints, collisions and rapid changes of direction (Akiyama et al., 2019; Polley et al., 2015). This high-intensity activity profile would be expected to lead to a rapid rise in core body temperature (Morris et al., 1998). In addition, because lacrosse is classified as a full-contact sport, players are required to wear protective equipment (shoulder pads, arm pads, gloves and helmet) during the match. However, wearing protective equipment is an important risk factor for the development of EHS (Casa et al., 2015), because it can inhibit evaporative, convective, and radiant heat loss (Casa et al., 2015), resulting in a faster rate of increases in core body temperature (Armstrong et al., 2010; Kulka et al., 2002; Osakabe et al., 2022). Although soccer players are not required to wear protective equipment, a previous study reported that peak core body temperatures exceeded 39.5°C during a soccer match in hot and humid conditions (36°C, 61% relative humidity [RH]) (Ozgünen et al., 2010). A recent study by Osakabe et al. (2022) reported that when wearing lacrosse uniforms during cycling exercise designed to mimic the activity patterns of a lacrosse match in hot conditions (35°C, 50% RH), the core body temperature at the end of the 4th quarter was 0.7°C higher than that when wearing general athletic clothes. Thus, lacrosse players are at a high risk of heat-related illness during the match due to the wear of protective equipment. However, options for external cooling strategies (e.g., wearing an ice vest) during lacrosse matches are limited because of the protective equipment worn by players.
Adequate hydration has long been considered one of the most important cooling strategies for reducing the risk of heat-related illness during exercise in hot conditions (Chalmers et al., 2020; Racinais et al., 2015). Following the example of the 2008 Olympics and the 2014 FIFA World Cup, additional recovery periods between and during competitions for hydration and body cooling opportunities have been recommended when competitions are held in hot conditions (Racinais et al., 2015). Addition of brief in-play cooling breaks is a feasible strategy for field-based continuous sports, and easily implemented at various levels (amateur to professional) (Chalmers, 2017). Therefore, several sporting associations (e.g., FIFA, Major League Soccer, A-league) have implemented additional in-play cooling time for competitions held in hot conditions. Since 2019, the Japan Lacrosse Association has implemented an additional in-play cooling time protocol called water break time out (WBTO) during matches held between July and September, which includes additional 2-min breaks at the 7-min mark of each quarter during the match (Japan Lacrosse Association, 2020). One recent study reported that 3-min of in-play cooling breaks with cold fluid ingestion attenuated the rise in core body temperature (rectal temperature) during a simulated soccer match in hot conditions (35°C, 55% RH) (Chalmers et al., 2019). Therefore, WBTO may provide a feasible and effective internal body cooling strategy during the match, even for players wearing protective equipment. In addition, for lacrosse players, WBTO may provide an opportunity to rest, perform additional fluid intake, and to remove some protective equipment, such as gloves and helmets, promoting evaporative and convective heat loss.
However, to the best of our knowledge, the cooling effects of the WBTO system implemented by the Japan Lacrosse Association during lacrosse matches in summer have not been examined. For reducing the risk of heat-related illness during the match, knowledge of the effects of WBTO on physiological responses is important for coaches, staff, and players. Thus, the purpose of the current study was to examine whether WBTO attenuated the core body temperature (rectal temperature) increase during intermittent cycling exercise that mimicked the activity patterns of a men’s lacrosse match while wearing a uniform in a hot-humid environment, compared with a regular match simulation condition. We hypothesized that implementation of WBTO during the intermittent cycling exercise would result in attenuation of the core body temperature rise compared with a regular match simulation condition.
Methods
Participants
Ten non-heat-acclimatized, physically active men (mean ± standard deviation; age=23±2 years, height=173.2±4.6cm, body mass [BM]=68.1±9.0kg, maximal oxygen uptake [VO2max]=48.9±7.8m L/kg/min, maximum heart rate [HRmax]=191±6 beats per min [bpm]) were recruited for the present study. Participants regularly completed more than two endurance and/or intermittent training sessions per week (≥30 min/session), but were not competitive athletes. All participants were healthy nonsmokers, with no history of heat-related illness or cardiovascular disease. Approval for this study was obtained from the Human Research Ethics Committee of the Chukyo University (2020-37) and written informed consent was obtained from all participants.
Design and procedures
All participants completed two experimental sessions in randomized, counterbalanced order, separated by at least 7 days to minimize carryover effects. In the simulated regular match (REG) condition, the experimental session consisted of a 15-min warm-up followed by 4×15-min intermittent cycling exercises separated by a 10-min simulated halftime break and 2×2-min quarter breaks (Figure 1a, total duration: 89min). In the WBTO condition, participants underwent the same exercise as in the REG condition, but were given additional 2-min breaks 7.5min into each quarter (Figure 1b, total duration: 97min) (Figure 1).
Figure 1
Experimental protocol in the REG (a) and WBTO (b) conditions.
VO2max: maximal oxygen uptake. 100 mL, 200 mL and 300 mL: amount of drink supplied.
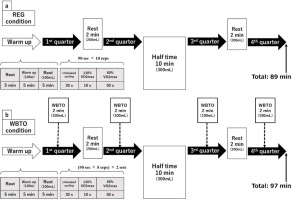
To simulate the environmental conditions of summer in Japan, all experimental sessions were performed in a climate chamber (TBR12A4PX; ESPEC, Osaka, Japan) set at 35°C, 50% RH, and 28°C Wet-Bulb Globe Temperature (WBGT). WBGT was measured using a WBGT monitor (AD-5695; A&D Co., Ltd., Tokyo, Japan). During all experimental sessions (excluding the warm-up [0–15 min] and half-time break), air flow (3 m/s) was provided from 1.5m in front of participants.
Participants were asked to maintain their normal lifestyle activities, including physical activity and nutritional habits, throughout the study period, and to refrain from strenuous physical activity, as well as alcohol, caffeine, and drug consumption, for 24h before all sessions. To control for circadian variations in body temperature, the two experimental sessions were implemented at the same time of day. To promote hydration prior to the start of each session, participants were also instructed to drink 500mL of plain water 2h before each session. All experimental sessions were implemented from January to March. In all experimental sessions, participants wore a sleeveless compression shirt (made of 100% polyester), jersey (for lacrosse; made of 100% polyester), shorts (for lacrosse; made of 100% polyester), ankle-length socks, athletic shoes, gloves, elbow guards, helmet, and a shoulder pad. The men’s lacrosse uniform used in this study was similar to the uniform generally used in the Japanese college lacrosse league.
Experimental protocol
On their first visit to the laboratory, participants performed a progressive exercise test on a cycle ergometer (Fujin-Raijin; O.C.Labo, Tokyo, Japan) in a climate chamber set to a temperate environment .(25°C and 50% RH) to determine the VO2max and HRmax. Prior to the test, the height and BM (MC-180; Tanita Co., Tokyo, Japan) of each participant were measured to the nearest 0.1cm and 50g, respectively. The incremental exercise test began with participants cycling at 100W for 5min, with 25W increases every 2min until volitional fatigue. Participants were required to maintain a pedal cadence of 80 revolutions per min (rpm) during the test. The test was considered to be valid if two of the following three criteria were met: (1) plateau in oxygen uptake, (2) heart rate (HR) remained within 10% of the predicted maximum (220−age), or (3) the respiratory exchange ratio was >1.05 (Stanley et al., 2010). Oxygen uptake and carbon dioxide production were measured by breath-by-breath gas analysis using an automatic gas analyzer (AE-310s; Minato Medical Science, Tokyo, Japan). The HR was continuously monitored during the test using a HR monitor (POLAR A300; Polar, Kempele, Finland).
Upon arriving at the laboratory on their second or the third visit for the experimental session, urine samples were collected and participants’ nude BM was measured. After placement of rectal and skin thermistors and the HR monitor, participants wore a sleeveless compression shirt, jersey, shorts, ankle-length socks, athletic shoes, elbow guards, and a shoulder pad. Next, participants entered a climate chamber, and rested on a chair for 5min, and then moved to the cycle ergometer, put on a helmet and gloves, and began a 5-min warm-up (100W, 80rpm). After the warm-up, participants rested again for 5-min, followed by the first 15-min intermittent exercise (1stquarter). The 15-min intermittent exercise consisted of 10 repetitions of a 90-s exercise period in the REG condition (Figure 1a), and two sets of five repetitions, each separated by a 2-min rest interval in the WBTO condition (Figure 1b). Each 90-s period started with 30s of unloaded cycling with a cadence of 80 rpm followed by 10s of high-intensity cycling (i.e., 130% of VO2max, 80 rpm), and ended with 50s of moderate-intensity cycling (i.e., 60% of VO2max, 80rpm). The 15-min intermittent exercise protocol was the same as that used in a previous study examining thermoregulation during cycling exercise designed to mimic the activity patterns of a lacrosse match (Osakabe et al., 2022), which was based on an earlier study designed to reflect a soccer match using a cycle ergometer (Yanaoka et al., 2018). The protocol was adjusted to match the activity profile of an actual lacrosse match reported in a previous study (Akiyama et al., 2018). After the 2ndquarter, participants were required to perform 1min of unloaded cycling at a self-selected pace to simulate walking to the locker room, then rested on a chair for 8min. At the end of the half-time break, participants performed 1min of unloaded cycling at a self-selected pace followed by 15-min of intermittent exercise (3rd quarter). After the 4th quarter, nude BM was measured after the rectal and skin thermistor and the HR monitor were removed, and urine samples were collected. During the experimental session, participants consumed a cold sports drink (4°C; Pocari Sweat; Otsuka Pharmaceutical, Tokyo, Japan) after the warm-up (100mL), after the 1stquarter (200mL), at half-time (300mL), and after the 3rdquarter (200mL). In the WBTO condition, participants consumed 100mL of cold sports drink at every additional break (400mL in total). To avoid gastrointestinal discomfort due to fluid ingestion, the amount of additional sports drink ingestion in the WBTO condition was adjusted and determined in pilot testing. In all experimental sessions, participants took off their helmets and gloves at pre and post warm-up, and in the quarter, half-time and additional breaks (in the WBTO condition).
Measurements
The environmental conditions (temperature and relative humidity) in the climate chamber were recorded before and after the experimental session, and averaged.
Urine specific gravity (USG) was measured before and after the experiment using a digital USG scale (PAL-09S, Atago, Tokyo, Japan) as an index of hydration status. To avoid dehydration before the experiment, a cutoff USG value of 1.020 was determined, and no participants exceeded this USG cutoff value before experiments. Nude BM was measured using a scale (MC-180; Tanita Co.) to the nearest 50g before and after the experiment. A rectal thermistor probe (LT-ST08-11; Gram Corporation, Saitama, Japan) was positioned approximately 15cm beyond the anal sphincter to measure rectal temperature (Tre). Skin thermistors (LT-ST08-12; Gram Corporation) were attached to the chest (Tchest), forearm (Tarm), thigh (Tthigh), and calf (Tcalf). Throughout the experimental sessions, rectal and skin temperature data were intermittently recorded using a data logger (LT-8; Gram Corporation) at 30-s intervals. The mean skin temperature (mTsk) was calculated using the following formula developed by Ramanathan (1964):
mTsk=0.3×(Tchest+Tarm)+0.2×(Tthigh+Tcalf)
The HR was measured using a HR monitor (POLAR A300; Polar) every 30 s throughout the experimental session. Percent maximum HR (%HRmax) was calculated using the following formula:
%HRmax=average HR of 1stquarter to the end of 4thquarter/HRmax×100
The physiological strain index (PSI) was calculated using the method reported by Moran et al. (1998):
PSI=5×(Tret−Tre0)/(39.5−Tre0)+5×(HRt−HR0)/(180− HR0),
where Tret and HRt are the values measured at a certain time point, Tre0 and HR0 are the values measured at the beginning of exercise, and 39.5 and 180 are the mean maximum values of core temperature and HR, respectively (Moran et al., 1998). The sweat rate was estimated using the following formula:
Sweat rate (kg/h)=(BM before the experiment[kg]−M after the experiment [kg]+the amount of the ingested drink [kg])/(exercise duration [h]).
Percent body mass loss was estimated using the following formula:
Percent body mass loss (%)=(BM before the experiment [kg]−BM after the experiment [kg]+the amount of the ingested drink [kg])/BM before the experiment [kg]×100
The rating of perceived exertion (RPE; 15-point scale) was recorded at each end of the quarter using the Borg scale (Borg, 1982). Thermal sensation (TS) was measured using a 9-point scale (1: very cold, 2: cold, 3: cool, 4: slightly cool, 5: neutral, 6: slightly warm, 7: warm, 8: hot, and 9: very hot) (Kashimura, 1986). Thermal comfort (TC) was measured using a 7-point scale (−3: very uncomfortable, −2: uncomfortable, −1: slightly uncomfortable, 0: neutral, 1: slightly comfortable, 2: comfortable, and 3: very comfortable). TS and TC were recorded at pre and post warm-up, before the 1stquarter, at the end of each quarter, and at the end of HT.
Statistical analyses
A priori sample size calculation was conducted using G*power (v3.1.9.7; Düsseldorf, Germany). Based on data from a previous study (Chalmers et al., 2019) that reported differences in the increase in Tre between a simulated regular soccer match in hot conditions and a simulated match with additional in-play cooling time, a minimum sample size of eight participants was considered to be required to demonstrate a difference in Tre (using a calculated effect size of 0.6, a power of 0.8, and an α-level of 0.05). All variables measured during the experiment are presented as mean ± standard deviation. All statistical analyses were performed using statistical software (SPSS v26.0; IBM Corp., Chicago, IL, USA). Data normality was tested using the Shapiro–Wilk test, and where there was a significant violation of sphericity, F values were adjusted using Greenhouse–Geisser or Huynh– Feldt corrections, as appropriate. A two-way repeated measures analysis of variance (condition × time) was used to analyze Tre, mTsk, HR, PSI, TS, TC, RPE, USG (n=10). The increases in Tre from baseline to each quarter and HT were also analyzed using a two-way repeated measures analysis of variance (condition × time). When a significant interaction was observed, post hoc analyses were performed using the Bonferroni correction. In this study, recorded values of Tre, mTsk, PSI and HR during the warm-up (0 – 15min) were defined as baseline values. Data for Tre, mTsk and PSI (mean of final 1-min) were analyzed at the end of each quarter and at the end of HT because the amount of time that elapsed was different between the two conditions. Data for the HR were averaged over each whole quarter (including WBTO) and HT, then analyzed. Differences in the sweat rate (estimated in 1.37h [97min] in the WBTO condition and 1.29h [89min] in the REG condition, respectively), post BM and percent body mass loss between the two experimental conditions were compared using a paired samples t-test. Differences were considered statistically significant at p<0.05. According to Cohen’s d, effect sizes (ES) were classified as small (0.2–0.5), moderate (0.5–0.8), or large (>0.8) (Cohen, 1988).
Results
The mean environmental conditions were 35.0±0.1°C room temperature and 49.5±1.0% relative humidity. The hydration states before and after the experiments are shown in Table 1. Post BM was significantly higher in the WBTO condition compared with the REG condition (p=0.011, d=0.45: small). There was no significant difference in the sweat rate (p=0.953, d=0.02: small), percent body mass loss (p=0.289, d=0.31: small) between the WBTO and REG conditions. No significant interaction between condition and time (p=0.149) was detected for USG.
Table 1
Hydration state before and after the experiment.
A significant interaction between condition and time (p=0.002) was detected for Tre. There was no significant difference in the baseline values of Tre between the WBTO and REG conditions (p=0.807, d=0.11: small). However, Tre was significantly lower in the WBTO condition (38.23±0.23°C) compared with the REG condition (38.50±0.46°C) at the end of the 4thquarter (p=0.017, d=0.69: moderate) (Figure 2).
Figure 2
Changes in rectal temperature during the experimental session in REG (○) and WBTO (▲). Values are expressed as means ± SD (n=0). 1st: at the end of the 1stquarter, 2nd: at the end of the 2ndquarter, 3rd: at the end of the 3rdquarter, 4th: at the end of the 4thquarter, HT: half-time.
* p<0.05 denotes a significant difference between the WBTO and REG conditions.
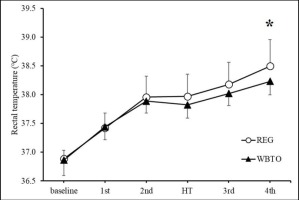
Increases in Tre from baseline to each quarter and HT are shown in Table 2.
Table 2
Thermal sensation, thermal comfort and changes in rectal temperature during the experimental session.
A significant interaction between condition and time (p = 0.002) was detected, however, post hoc testing revealed that the increase in Tre was not significantly different between WBTO and REG conditions at any time points. The increase in Tre from baseline to the end of the 4thquarter was 1.61±0.43 and 1.37±0.37 in REG and WBTO conditions, respectively (p=0.077, d=0.57: moderate). There was no significant interaction in mTsk between the WBTO and REG conditions (p=0.299). The baseline values of mTsk were 34.07±0.49°C and 34.10±0.48°C in the WBTO and the REG condition, respectively, and reached 36.22±0.80°C in the WBTO condition and 36.63±0.73°C in the REG condition at the end of the 4thquarter, respectively. A significant main effect of condition (p=0.009) was detected for the HR. The HR was significantly lower in the WBTO condition compared with the REG condition during the experimental trials. The baseline values of the HR were 74±11bpm and 79±14bpm in the WBTO condition and REG condition, respectively, and reached 150±10bpm in the WBTO condition and 159±11bpm in the REG condition at the end of the 4thquarter. Mean %HRmax during experimental sessions in the REG condition was 75±6%. A significant interaction between condition and time (p=0.001) was detected for PSI. PSI was significantly lower in the WBTO condition (6.5±0.9) compared with the REG condition (7.2±1.4) at the end of the 4thquarter (p=0.043, d=0.59: moderate).
A significant interaction between condition and time (p = 0.022) was detected for the RPE. The RPE was significantly lower in the WBTO condition compared with the REG condition at the end of the 3rdquarter (p=0.001, d=2.12: large) to the end of the 4thquarter (p=0.002, d=1.53: large), and reached 14.3±1.6 in the WBTO condition and 16.6±1.2 in the REG condition at the end of the 4thquarter. A significant interaction between condition and time (p=0.005) was detected for TS. TS was significantly lower in the WBTO condition compared with the REG condition at the end of the 2ndquarter (p=0.037, d=0.80: moderate) and at the end of the 4thquarter (p=0.04, d=0.72: moderate) (Table 2). There was no significant difference in TC between the WBTO and REG conditions (Table 2; p=0.81).
Discussion
The purpose of the current study was to examine whether implementing 2-min WBTO in each quarter would attenuate the rectal temperature rise during a cycling intermittent exercise protocol designed to mimic the activity pattern of a men’s lacrosse match in a hot-humid environment compared with a regular match simulation. In accordance with our hypothesis, the WBTO condition significantly attenuated the rise in Tre compared with the REG condition, but this attenuation only occurred in the latter stages of the cycling intermittent exercise protocol designed to mimic the activity pattern of a men’s lacrosse match. In addition, we found that HR, PSI, RPE and TS were significantly lower in the WBTO condition compared with the REG condition.
We found that implementing 2-min of additional WBTO in each quarter significantly attenuated the core body temperature elevation (Tre) only in the latter stages of the cycling intermittent exercise protocol designed to mimic the activity pattern of a men’s lacrosse match in a hot-humid environment. The difference in Tre at the end of the 4th quarter was 0.27°C (WBTO: 38.23±0.23°C; REG: 38.50±0.46°C). These differences in absolute core temperature are physiologically meaningful because the magnitude in reduction of core temperature is similar to the effects of heat acclimation (Tyler et al., 2016) and pre-cooling (Bongers et al., 2015). A previous study (Chalmers et al., 2019) reported that additional 3-min cooling periods (i.e., cold water ingestion [10°C; 3.2mL/kg]) in each half during a simulated soccer match in a hot-humid environment significantly attenuated the rise in Tre compared with a simulated regular match (no additional cooling period), and the difference in Tre at the end of exercise was 0.25°C. Thus, the current results are consistent with this previous study. In the current study, although the increases in Tre from baseline to the end of the 1st, 2nd, HT and 3rd quarter were not significantly different between conditions, the increases in Tre from baseline to the end of the 4th quarter in the WBTO condition (1.37±0.37°C) tended to be lower (p=0.077) than those in the REG condition (1.61±0.43°C) (Table 2). These results suggest that, although the cooling effect of a single implementation of WBTO was small, the cumulative cooling effect of WBTO increased with repeated implementation in each quarter. In lacrosse competition matches, the use of external body cooling strategies, such as wearing cooling jackets, is limited, because players cannot remove protective equipment during the match. However, despite the lacrosse uniforms worn by participants in the current study, the implementation of WBTO, as recommended by the Japan Lacrosse Association, was associated with lower Tre only in the latter stages of the experimental session. Although the cooling effects of WBTO on Tre were observed only in the latter stages of an intermittent cycling exercise protocol designed to mimic the activity pattern of a men’s lacrosse match, WBTO appears to provide a feasible cooling strategy for players at various levels (amateur to professional) in lacrosse matches and/or practice games in hot-humid environments.
In addition, we found that PSI was significantly reduced at the end of the 4th quarter in the WBTO condition compared with the REG condition (WBTO:6.5±0.9; REG:7.2±1.4). PSI is based on rectal temperature and HR, and was developed to evaluate heat stress at an individual level (Moran et al., 1998). Physiological strain is described on a universal scale of 0–10, where 0 represents “no strain” and 10 “very strenuous” (Moran et al., 1998), while >7.5 is categorized as “at risk” for heat-related illness (Buller et al., 2008). A recent study by Osakabe et al. (2022) reported that mean PSI values exceeded 7.5 from the latter stages of the 3rd quarter during the cycling intermittent exercise protocol designed to mimic the activity pattern of a men’s lacrosse match in a hot-humid environment. In the current study, the PSI value at the end of the 4th quarter in the REG condition (7.2±1.4) was very close to the critical marker of 7.5, whereas the PSI value was considerably below the critical marker of 7.5 in the WBTO condition (6.5±0.9). Thus, our data suggest that the implementation of WBTO can be an effective cooling strategy for reducing physiological strain during lacrosse matches in hot-humid conditions, only in the latter stages of the match when the risk of heat-related illness is high.
The RPE and TS were significantly improved by implementing WBTO during the cycling intermittent exercise protocol designed to mimic the activity pattern of a men’s lacrosse match in a hot-humid environment. A previous study also reported that the RPE and TS were significantly improved by the implementation of additional 3-min cooling periods (i.e., cold water ingestion [10°C; 3.2mL/kg]) in each half during a simulated soccer match in a hot-humid environment (Chalmers et al., 2019). Improvements in TS during the cycling intermittent exercise protocol may have been caused by limiting exercise during additional breaks in each quarter, and players having the opportunity to take off their gloves and helmet. These additional breaks might also contribute to reduction in the HR because players have an opportunity to rest. The measurement of the HR is strongly related to the RPE, and the lower RPE in the WBTO condition is likely to be mediated by improvements in the HR. Thus, our data suggest that the implementation of WBTO may provide an effective cooling strategy for reducing perceptual strain during the cycling intermittent exercise protocol designed to mimic the activity pattern of a men’s lacrosse match.
The present study involved several potential limitations. First, lacrosse players are required to perform repeated running sprints and changes of directions in an actual match, and unlimited interchanges are allowed (Akiyama et al., 2019; Polley et al., 2015). Average %HRmax during intermittent cycling exercise (75%; including all rest breaks) in the current study was slightly lower than that reported in an actual lacrosse match (78%; Akiyama et al., 2019). Therefore, the actual exercise intensity during lacrosse (running) is likely to be higher than that in the present study (cycling). Because of differences in the metabolic rate of muscle activity between running and cycling, greater thermal responses are potentially induced in the actual match. It was previously reported that soccer players had a core temperature in excess of 39.5°C during a soccer match in hot and humid conditions (Özgünen et al., 2010). In contrast, Tre in the current study stayed below 38.5°C. Thus, although our exercise protocol was designed to mimic activity patterns of a men’s lacrosse match using a cycle ergometer, it was difficult to accurately replicate the conditions of an actual match and then evaluate the cooling effects of WBTO at a high core body temperature (> 39.0°C) which would involve a high risk of heat-related illness. Second, to minimize the effects of dehydration on thermal and perceptual responses between two conditions in this study (i.e., percent BM loss was −2.1% in the REG condition and −2.2% in the WBTO condition), the amount and timing of fluid ingestion in this study were planned. However, in actual matches, players ingest fluid as needed, ad libitum. Therefore, the effects of WBTO with ad libitum fluid ingestion on thermal and perceptual responses remain unclear. Overall, further studies are warranted to investigate the cooling effects of WBTO during actual lacrosse matches held in hot-humid environmental conditions.
Conclusions
Even while wearing a lacrosse uniform, the implementation of WBTO attenuated the rise in Tre only in the latter stages of the intermittent cycling exercise protocol designed to mimic the activity pattern of a men’s lacrosse match in hot-humid conditions. In addition, the implementation of WBTO attenuated physiological (PSI, HR) and perceptual strain (RPE, TS). These results suggest that although further research is needed to investigate the cooling effects of WBTO in the actual lacrosse match, the current study supports the use of additional in-play cooling breaks (WBTO) recommended by the Japan Lacrosse Association during lacrosse matches in hot-humid environments. Additional in-play cooling breaks may provide a feasible and effective body cooling strategy for amateur to professional level lacrosse to reduce the risk of heat-illness.